The effect of crocin on neuroprotective activity in vitro: a narrative review
Introduction
Crocus sativus L. belonging Iridaceae cultivation was recorded in Crete 3,500 years ago, and it has been expanding in Iran, Greece, Spain, Morocco, China and Japan, for its red stigma (Figure 1).
C. sativa flowers once in a year and its stigma should be harvested during very short period. Therefore, saffron is too expensive comparing with the other herb medicines.
Crocetin glycosides, picrocrocin, and safranal are the major components of saffron (Figure 2). Among them, crocin concentration is around 15 % or more (1,2). Crocin 2, 3 and 4 are contained as the minor constituents.
Since indoor cultivation systems were started in Japan from 1910 (1) because the quality of saffron depends on weather conditions (Figure 3). Indoor cultivation systems have several benefits such as adjusting full blooming period resulting in labor reduction. Moreover the quality control of saffron is improved since saffron contains strong β-glucosidase which cleavages glycoside linkage to give crocin 2, 3 and 4 as shown in Figure 2 under moisture condition (1). This is the reason why the short time drying of saffron is needed. The relation between biological activity and sugar number conjugated was reported that the saponin’s haemolytic activity of di- and triglycoside saponins were higher than that of monoglycoside (3). From this evidence, it is easily suggested that the higher glycoside of crocetin, crocin might possess higher activity. This is an important issue for the quality control of saffron (4). Therefore, dried saffron is then frozen and stored free of moisture to preserve its high quality (1).
We present the following article in accordance with the Narrative Review reporting checklist (available at https://lcm.amegroups.com/article/view/10.21037/lcm-22-4/rc).
Methods
Studies published over the last 20 years were identified via a PubMed search using different combination such as “Crocus sativus”, “Saffron”, “Crocin”, “neuroprotective”, “PC-12 cell”, “apoptosis”. Most of search formulas were shown in Table 1. The search strategy was summarized in Table 2.
Table 1
("Crocus sativus"[Mesh]) AND "neuroprotective"[Mesh] |
("Saffron"[Mesh]) AND "neuroprotective"[Mesh] |
("Crocin"[Mesh]) AND "neuroprotective"[Mesh] |
("Crocus sativus"[Mesh]) AND "PC-12 cell"[Mesh] |
("Saffron"[Mesh]) AND "PC-12 cell"[Mesh] |
("Crocin"[Mesh]) AND "PC-12 cell"[Mesh] |
("Crocus sativus"[Mesh]) AND "apoptosis"[Mesh] |
("Saffron"[Mesh]) AND "apoptosis"[Mesh] |
("Crocin"[Mesh]) AND "apoptosis"[Mesh] |
Table 2
Item | Specification |
---|---|
Date of search | 2021/12/15–2022/02/20 |
Database and other sources searched | PubMed |
Search terms used | Details in Table 1 |
Time frame | 1980–2021 |
Inclusion and exclusion criteria | Inclusion criteria: research articles and reviews in English about themes such as Crocus sativa/saffron/crocin and neuroprotection/PC-12 cell/apoptosis |
Exclusion criteria: some papers which we considered with low reliability | |
Selection process | Yukihiro Shoyama conducted the selection, authors attended a discussing the literature selection and obtained the consensus |
Any additional consideration | Some papers were identified by reviewing reference lists of relevant publications if applicable |
Additional papers are identified by reviewing reference lists of publications. Publications with relative low credibility and publications without English are removed. Data are extracted depending on justness for the topic and its detail are shown in Table 2.
Saffron was listed in the Bencao Gangmu published in China in 1578 for its cognitive functions, and has been known for its blood disorder (5), anti-cancer (6-8), anti-oxidant activity (9,10), memory impairment (11-15), learning and memory (12,15,16) which are closely related to dementia. Brain dysfunction models mimic the protection of neuronal function such as in anti-amyloid-βaggregation (17), NMDA receptor (13,18). Naghibi et al. investigated the effect of saffron extract on morphine-induced memory impairment (19). The number of sugars in crocetin glycosides reflected the ethanol-mediated LTP inhibition. Crocin showed the highest activity comparing with crocin 2, 3 and 4. This evidence is consistent with previous reports which sugar numbers are important for the activities of saponins such as cardiac glycosides (20), ginsenosides (21), saikosaponins (22) and hemolytic saponins (3,23). Recently natural products preventing dementia is strongly desired under the rapid increase of dementia patients. With respect to the safety of natural products, the most important factor for investigation is the confirmation of no toxicity of the major constituents. Saffron is chosen in this review since it has been used from approximately 3,000 years ago as a spice, coloring and medicine recorded in materia medica 2,000 years ago. In the case of health volunteers saffron (200 and 400 mg) are safe drug on coagulation system under a double-blind, placebo-controlled study for 1 week (24). When old AD patients were administered saffron extractives (30 mg) or donepezil (10 mg) as a positive control under a double-blinded/phase II study, the effect of saffron extract was evaluated to be the same with donepezil for mild to moderate AD patients. The major adverse effect of saffron, vomiting was lower than that of donepezil (25). Volunteers received crocin tablet (20 mg) or placebo resulted that the crocin tablet showed no major side effect except decreases of amylase, white blood cells and partial thromboplastin time (26). Therefore, the neuroprotective activities of saffron and crocin will be incorporated in the current study. Regarding the neuroprotective activity of saffron related to dementia, several processes were associated including enhancing nerve growth factor (NGF) secretion (27), choline acetyltrasferase (ChAT) activity and its mRNA levels (28), inhibition of β-amyloid secretion (29), promotion of energy metabolism in neurons via protection of mitochondrial damage (30) and sirtuin 1 (SIRT1) protein activation (31,32). Among these pathways, Crowe et al. reported that programmed cell death (apoptosis) in neurons occurs in the brain deprived of oxygen after stroke (33,34), as well as in patients with Alzheimer’s disease (35). The prevention of neuronal apoptosis might become a promising therapeutic strategy for a neurodegenerative disease. The neuronal cell death, apoptosis was occurred by many pathways and their mechanisms will be confirmed in this review. Among them anti-oxidative stress, γ-glutamyl cycle promotion and inhibition of ceramide release which were found firstly by authors, and the proteins related to memory were included in this review. The wide field data related to the neuronal investigations has been accumulated by the rat pheochromocytoma (PC-12) cells easily differentiated into neuron-like cells. Therefore, the neuroprotective activity of crocin using PC-12 cells is reviewed.
Preparation of anti-crocin monoclonal antibody and immunostaining of PC-12 cells
Crocin possessing 4 glucoses in a molecule in saffron is unstable because saffron contains inner β-glycoside resulting in hydrolysis occurrence under moisture condition (1). Moreover, the polyene structure in crocin is unstable under the existence of oxygen. From these reasons the quality control of saffron and/or crocin is needed before research. In our ongoing research of monoclonal antibody (MAb) for natural products the preparation of anti-crocin MAb has been started.
In order to prepare MAb against crocin the conjugate of crocin and carrier protein is necessary. Synthesis of crocin-carrier protein conjugate has two pathways because crocin possess 4 glucoses in a molecule, one is the cleavage method by NaIO4 to prepare aldehyde body which can be conjugated with carrier protein via shiff’s base bond as prepared for many MAbs against saponins like ginsenosides (36,37), paeoniflorin (38), saikosaponin (39), glycyrrhizin (40) and so on. In the first stage of MAb preparation the exact structure of crocin-carrier protein is unknow because crocin has many cleavage points by NaIO4. As the second method a hydroxyl group on C-6 of terminal glucose was specifically reacted with succinate anhydrate to give a hemisuccinate which combined with carrier protein producing crocin carrier protein conjugate. The hapten number in conjugate was determined by MALDI-tof-MS. When compared the hapten numbers prepared by NaIO4 and hemisuccinate pathway, respectively the later method was clearly higher than that of NaIO4 method (41). Crocin-hemisuccinate-HAS conjugate was used because the higher hapten number in the conjugate promoted the preparation of MAb (42) and prepared MAb against crocin by usual way via hypoxanthine- aminopterin-thymidine (HAT)-sensitive mouse myeloma cell (43,44). Single cell cloning by limited dilution way (45) was succeeded vir HAT-selection and screening with direct enzyme linked immuno solvent assay (ELISA) (42). The cross-reactivity was not specific for crocin, for example 66.9%, 61.6% and 1.5% for crocin 2, crocin 3 and crocin 4, respectively. However, it did not react with monoterpenoids, carotenoids and saponins. This wide reactivity is the main advantage of the antibody. It is better than a specific antibody for the metabolic study on crocin and the study of pharmacologically active mechanism of crocin in the central nervous system because it is suggested this MAb should be helpful to the further study on the presumable receptor in the brain.
To verify the incorporation of crocin and its localization in PC-12 cells, cells were immunostained using the anti-crocin MAb. Figure 3 presents the time course of 10 µM crocin in PC-12 cells over a 30 min time span. Clear incorporation of crocin after incubation of 30 and 60 min into PC-12 cells was confirmed in comparison with control cells as presented in Figure 3. Incorporation of crocin in cells was recently confirmed by bone marrow mesenchymal stem cells (46) and plant cells (47) too. From these findings the incorporation of crocin into cells was exactly confirmed by anti-crocin MAb (9). It is easily suggested that transformation and/or metabolism of crocin will be occurred in cultured PC-12 cells because it became clear that alpha-glycosyl rutin, higher soluble rutin is hydrolyzed in vitro (48). Fortunately, the prepared anti-crocin MAb can cover the metabolites if it will be occurred in neuronal cells. Immunostaining using the anti-crocin MAb confirmed its incorporation and localization of crocin in PC-12 cells (9) as shown in Figure 4 resulted that PC-12 cells can be used for further neuronal investigation together with anti-crocin MAb such as the immunostaining analysis for several proteins related to neuroprotective activity in PC-12 cells similar to that Li et al. reported the immunostaining of Cx36 and CaMKII in the investigation of neuroprotective natural product, leonurine in PC-12 cells (49).
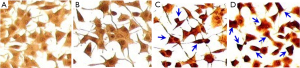
Inhibitory activity of crocin against PC-12 cell death induced by serum/glucose deprivation (SGD) medium
PC-12 cells have been used widely for the neuronal investigation because it is easily differentiated into neuron-like cells. Furthermore, the clear cell death is induced by serum and/or glucose deprivation in culture medium.
Ochiai et al. found that 60% cell death was occurred after 1 day of serum/glucose deprivation (50). This phenomenon was due to apoptotic cell death via the oxidative stress induced by the increase of malonyldialdehyde (MDA) and the decrease of glutathione (GSH) levels resulting in the activation of caspase-3, -8, and -9, and increased Box levels with the decreases of Bcl-2 levels and NF-κB binding activity (48). Moreover, serum deprivation was linked with the deactivation of mitochondrial function, like confirmed by cytosolic release of cytochrome c and JC-1 (51). Glucose deprivation caused apoptosis in HeLa cell, which was prevented by knockdown of caspase-8 resulting in caspase-8-driven and receptor-independent apoptosis with no occurrence of mitochondrial outer membrane permeabilization (MOMP) (52). From this evidence we confirmed that serum/glucose deprivation (SGD) medium can be used for the evaluation of activity for crocin using PC-12 cells.
Cultured cells in Dulbecco’s modified Eagle’s medium contained serum and glucose showed a normal morphology at 24 h. When cells were cultured in the SGD medium for 24 h, the apoptotic cells such as rolling shape were observed. It became clear that approximately 60% of cell death were determined by the Trypan blue dye method (50).
The addition of crocin (10 µM) significantly inhibited the morphological changes indicated that crocin blocked TNF-α-induced PC-12 cell death (53) showing 85% surviva1 compared to control cells. It is well known that serum deprivation gives apoptosis in PC-12 cells (54-56). Colombaioni et al. found that serum deprivation increased intracellular ceramide levels in undifferentiated HN9.10e cells inducing apoptosis. This evidence suggests that ceramide concentration increase in PC-12 cel1s incubated in SGD medium. When PC-12 cells are cultured in SGD medium for 3 h, ceramide increase significantly (3.5-fold) compared to the cells cultured in SGE medium (57). The addition of crocin inhibited PC-12 cell death dose-dependently. Verheij et al. postulated that the SAPK/JNK signaling systems and the sphingomyelin (SM) pathway formed a mixed function in apoptotic phenomenon induced by stress in U937 cells and BAE cells (58). It becomes evident that environmental stress under SGD conditions activates the stress-activated protein kinase (SAPK)/JNK cascade in PC-12 cells when compare the level of phosphorylated JNK in cells cultured in SGD medium and positive medium for 6 h. In fact, the SGD medium stimulates JNK phosphorylation in PC-12 cells approximately 4-fold comparing with control cells (58).
Antioxidant effect of crocin against neuronal cell death
A large number of investigations has been focused on antioxidant plants and their constituents. Among them, the antioxidant activities of saffron and crocin were investigated by many groups (59-68). Ochiai et al. also investigated the antioxidant activity of crocin using different assay system and the effects of crocin on PC-12 cells in DSG medium comparing with a-tocopherol (9). PC-12 cells were cultured in SGD medium resulted that morphological changes including membrane lipids were occurred whereas intracellular superoxide dismutase (SOD) activity was confirmed to be decrease by survey of Annexin V staining (Figure 5). Phosphatidyl serine (PS) residues are unusually sticked to the inner membrane, however under oxidative stress PS externa1ization appears as an ear1y stage of apoptosis. Since the negative1y charged PS attaches to Annexin, a ring-1ike staining occurs by conjugation with fluorescein isothiocyanate (FITC). When PC-12 cells are cultured in secoisolariciresinol diglucoside (SDG) medium, clear ring-1ike staining appears comparing with control cells. Crocin showed the more intact cell morphology than that of α-tocopherol. PC-12 cells cultured in SDG medium for 6 h indicated a 1.8-fold increase of peroxidized membrane lipid contents and a 14-fold increase of SOD activity comparing with control cells. On the other hand, crocin significantly inhibited the formation of peroxidized membrane lipids and the SOD activity compared with the α-tocophero1 activity as shown in Figure 5. The decrease of SOD activity indicated that crocin has an important duty for modu1ating antioxidative activities and for inhibiting the activation of caspase-8 released by DSG medium concentration-dependently in the concentration of 0.1–10 µM. Crocin did not affect caspase-8 activity in cell lysates and its inhibitory effects might be indirectly mediated by its antioxidant activities (9).
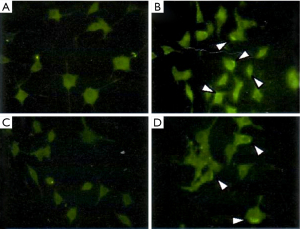
Increase of intracellular glutathione levels in serum glucose deprivation PC-12 cells through an increase in the activities of glutathione reductase and c-glutamyl cysteinyl synthase by crocin
It was known that the decrease of glutathione (GSH) levels gave apoptosis by the oxidative stress (69). GSH levels in PC-12 cells cultured for 3 h in SGD medium reduced to approximately half comparing with control cells and kept constant then after. However, the addition of crocin in the medium raised levels of intracellular GSH dose-dependently and its level continued for 3 h. A 10 µM of crocin indicated the highest levels of intracellular GSH. The GSH levels were satisfactory for inactivation of N-SMase. GR activities of PC-12 cells in the SGD medium reduced time dependently, however a 10 µM crocin addition promoted the activity of GR around a 4-fold increase at 6 h. This finding shows that crocin has no major outcome on the GPx activity in cells. GSH synthesis is regulated by the rate-limiting enzyme c-GCS which may be controlled by different pathways. TNF-α and IL-lβ promote c-GCS activity associated with an increase of mRNA expression in mouse endothelial cells. IL-6 also induces the expression of c-GCS mRNA and increases its activity, reaching to increased GSH levels in PC-12 cells. NGF accelerated the c-GCS activity at the transcription level by prolonging the half-1ife of c-GCS mRNA. Addition of 10 µM crocin promoted a two time increase of c-GCS mRNA expression in PC-12 cells incubated in SGD medium although it did not affect on the mRNA levels of control PC-12 cells. The crocin-mediated increase in expression of c-GCS mRNA relates an increase of this enzyme activity in cells. From these findings it is suggested that crocin can increase the levels of GSH by increasing the incubation of DMEM (50).
Proteins in central nervous system
The neuroprotection was associated with several proteins and/or enzymes such as acetylcholine esterase inhibition (28), inhibition of β-amyloid secretion (29) and improving β-amyloid-induced behavioral damage (70-73), ERK (74), AMPA receptor (74), NMDA receptor (13), CaMKII (74,75), promotion of energy metabolism in neurons via protection of mitochondrial damage (30) and SIRT 1 protein activation (31,32). We found that LTP induced by strong tetanic stimulation in the presence of 20 µM crocin and 75 µM ethanol was significantly bigger than that of the presence of 75 µM ethanol alone (12). This phenomenon indicated that crocin attenuated the action of ethanol. The strength of LTP was proportional to the sugar numbers conjugated to crocetin indicating that crocin was significantly strong, and reduced to crocin-2, -3 and -4 (15). This tendency is the same with saponins such as ginsenosides (21), saikosaponins (22) and cardiac saponin (20). Gene disruption of CaMKII in animal clearly inhibited memory and LTP occurrence resulted that CaMKII and LTP are closely related (76). Moreover, serious intellectual disability in human being was occurred by the genetic mutation of CaMKII (77). Therefore, the relation between LTP and CaMKII was suggested that the induction of Ca++ into cells activated CaMKII of which Ca++ moved to synapse and bound to the NMDA receptors and prepared LTP by mainly phosphorylating and accomplice subunits of AMPA receptors. Adabizadeh et al. investigated that crocin improved spatial memory inhibited by hyoscine, and NMDA, AMPA, ERK and CaMKII were analyzed by Western blot resulting in no change except ERK. The authors suggested that crocin only affected the elevation of ERK level in rat hippocampus (74). However, Chang et al. found that CaMKII was activated but the stimulation term was very short just about 1 min (78). From these evidence, the change of CaMKII stimulated by crocin can not be detected contrary LTP continued for 1 hr or more (13). Learning/memory and LTP behaviors are detected easily, but the transmitter and/or enzyme reaction are sometimes no detection although they are closely associated together. So that it is needed to confirm the pharmacological activity and the enzymatic activity and/or the transmitter activity together.
Conclusions
Saffron possesses a wide spectrum of pharmacological activities as crocin increases the intracellular glutathione levels and prevents cell death in serum-deprived and hypoxic PC-12 cells by its antioxidant properties. The antioxidant potential of crocin has been previously described in association with a variety of neuroprotective properties. In PC-12 cells, generation of ROS activates neutral SMase to generate ceramide resulting in cell death while glutathione directly inhibits the activation of the SMase. It is hypothesized that crocin could prevent the activation of N-SMase in PC-12 cells growing in SGD medium by a GSH-dependent inhibition mechanism. Ceramide release activates the caspase family as previously discussed as shown in Figure 6.
As discussed previously the neuronal enzyme reaction related to for example LTP is sometimes no detection although they are closely related. This evidence indicated that both in vitro and in vivo investigations are necessary for understanding real function of crocin. Therefor it is needed to confirm the pharmacological activity together.
We have also discovered that crocin can have an effect on cerebral infarction area caused by middle cerebral artery (MCA) occlusion in mice as indicated in Figure 7 (10). It is suggested that apoptosis causes neuronal cell death after acute brain ischemia although the mechanism is not completely evident (79). This evidence also supports the correlation of saffron and/or crocin with brain health supported by that crocin prevented neuronal cell death in vitro as described previously and MCA in mice via neuronal cell death which may be started from apoptosis in vivo. As previously discussed crocin prevented neuronal cell death in vitro and we found the prevention of MCA by administration of crocin in mice. This result suggested that the combination of in vitro investigation which may cover a wide range of mechanism and in vivo experiments which shows the exact phenomena is necessary. From this suggestion we reviewed in vitro, in vivo and clinical trial related to neurological disorders using saffron and/or crocin.
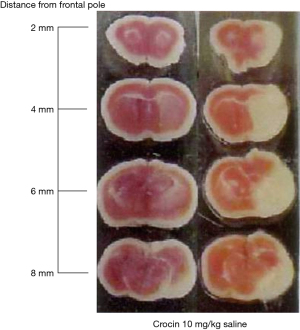
Acknowledgments
The authors sincerely thank the Faculty of Pharmacy of Nagasaki International University for providing the facilities to support this project and the Association for Health Economics Research and Social Insurance and Welfare for financial support.
Funding: None.
Footnote
Provenance and Peer Review: This article was commissioned by the editorial office, Longhua Chinese Medicine for the series “Multifunctional Saffron”. The article has undergone external peer review.
Reporting Checklist: The authors have completed the Narrative Review reporting checklist. Available at https://lcm.amegroups.com/article/view/10.21037/lcm-22-4/rc
Peer Review File: Available at https://lcm.amegroups.com/article/view/10.21037/lcm-22-4/prf
Conflicts of Interest: All authors have completed the ICMJE uniform disclosure form (available at https://lcm.amegroups.com/article/view/10.21037/lcm-22-4/coif). The series “Multifunctional Saffron” was commissioned by the editorial office without any funding or sponsorship. YS served as the unpaid Guest Editor of the series. The authors have no other conflicts of interest to declare.
Ethical Statement: The authors are accountable for all aspects of the work in ensuring that questions related to the accuracy or integrity of any part of the work are appropriately investigated and resolved. This article is not concerned with any animal investigation and clinical trial, only limited to publication analysis.
Open Access Statement: This is an Open Access article distributed in accordance with the Creative Commons Attribution-NonCommercial-NoDerivs 4.0 International License (CC BY-NC-ND 4.0), which permits the non-commercial replication and distribution of the article with the strict proviso that no changes or edits are made and the original work is properly cited (including links to both the formal publication through the relevant DOI and the license). See: https://creativecommons.org/licenses/by-nc-nd/4.0/.
References
- Morimoto S, Umezaki Y, Shoyama Y. Post-harvest degradation of carotenoid glucose esters in saffron. Planta Med 1994;60:438-40. [Crossref] [PubMed]
- Gregory MJ, Menary RC, Davies NW. Effect of drying temperature and air flow on the production and retention of secondary metabolites in saffron. J Agric Food Chem 2005;53:5969-75. [Crossref] [PubMed]
- Voutquenne L, Lavaud C, Massiot G, et al. Structure activity Relationships of Haemolytic Saponins. Pharm Biol 2002;40:253-62. [Crossref]
- Marieschi M, Torelli A, Bruni R. Qauality control of saffron (Crocus sativus L.): development of SCAR markers for the detection of plant adulterants used as bulking agents. J Agric Food Chem 2012;60:10998-1004. [Crossref] [PubMed]
- Lee IA, Lee JH, Baek NI, et al. Antihyperlipidemic effect of isolated from the fructus of Gardenia jasminoides and its metabolite crocetin. Biol Pharm Bull 2005;28:2106-10. [Crossref] [PubMed]
- Aung HH, Wang CZ, Ni M, et al. Crocin from Crocus sativus possesses significant anti-proliferation effects on human colorectal cancer cells. Exp Oncol 2007;29:175-80. [PubMed]
- Chryssanthi DG, Lamari FN, Iatrou G, et al. Inhibition of breast cancer cell proliferation by style constituents of different Crocus species. Anticancer Res 2007;27:357-62. [PubMed]
- Kawabata K, Tung NH, Shoyama Y, et al. Dietary Crocin Inhibits Colitis and Colitis-Associated Colorectal Carcinogenesis in Male ICR Mice. Evid Based Complement Alternat Med 2012;2012:820415. [Crossref] [PubMed]
- Ochiai T, Ohno S, Soeda S, et al. Crocin prevents the death of rat pheochromyctoma (PC-12) cells by its antioxidant effects stronger than those of α-tocopherol. Neurosci Lett 2004;362:61-4. [Crossref] [PubMed]
- Ochiai T, Shimeno H, Mishima K, et al. Protective effects of carotenoids from saffron on neuronal injury in vitro and in vivo. Biochim Biophys Acta 2007;1770:578-84. [Crossref] [PubMed]
- Zhang Y, Shoyama Y, Sugiura M, et al. Effects of Crocus sativus L. on the ethanol-induced impairment of passive avoidance performances in mice. Biol Pharm Bull 1994;17:217-21. [Crossref] [PubMed]
- Sugiura M, Shoyama Y, Saito H, et al. The effects of ethanol and on the induction of long-term potentiation in the CA1 region of rat hippocampal slices. Jpn J Pharmacol 1995;67:395-7. [Crossref] [PubMed]
- Abe K, Sugiura M, Shoyama Y, et al. Crocin antagonizes ethanol inhibition of NMDA receptor-mediated responses in rat hippocampal neurons. Brain Res 1998;787:132-8. [Crossref] [PubMed]
- Saito H, Sugiura M, Abe K, et al. Effects of ethanol extract of Crocus sativus L. and its components on learning behavior and long-term potentiation. Studies In Natural Products Chemistry. Elsevier 2001:955-69.
- Sugiura M, Shoyama Y, Saito H, et al. Crocin (crocetin di-gentiobiose ester) prevents the inhibitory effect of ethanol on long-term potentiation in the dentate gyrus in vivo. J Pharmacol Exp Ther 1994;271:703-7. [PubMed]
- Akbari F, Moghadasi M, Mohammad A, et al. The Effect of Eight Weeks Moderate-Intensity Endurance Training With Saffron Intake on Memory and Learning in Rats With Trimethytin Model of Alzheimer’s Disease. J Appl Exer Physiol 2019;15:115-28.
- Papandreou MA, Kanakis CD, Polissiou MG, et al. Inhibitory activity on amyloid-beta aggregation and antioxidant properties of Crocus sativus stigmas extract and its crocin constituents. J Agric Food Chem 2006;54:8762-8. [Crossref] [PubMed]
- Lechtenberg M, Schepmann D, Niehues M, et al. Quality and Functionality of Saffron: Quality Control, Species Assortment and Affinity of Extract and Isolated Saffron Compounds to NMDA and σ 1 (Sigma-1) Receptors. Planta Med 2008;74:764-72. [Crossref] [PubMed]
- Naghibi SM, Hosseini M, Khani F, et al. Effect of Aqueous Extract of Crocus sativus L. on Morphine-Induced Memory Impairment. Adv Pharmacol Sci 2012;2012:494367. [Crossref] [PubMed]
- Shimada K, Ishii N, Ohishi K, et al. Structure-activity relationship of cardiac steroids having a double linked sugar and related compounds for the inhibition of Na+, K+-adenosine triphosphatase. J Pharmacobio Dyn 1986;9:755-9. [Crossref] [PubMed]
- Takemoto Y, Ueyama T, Saito H, et al. Potentiation of nerve growth factor-mediated nerve fiber production in organ cultures of chicken embryonic ganglia by ginseng saponins: structure-activity relationship. Chem Pharm Bull (Tokyo) 1984;32:3128-33. [Crossref] [PubMed]
- Abe H, Sakaguchi M, Konishi H, et al. The effects of saikosaponins on biological membranes. 1. The relationship between the structures of saikosaponins and haemolytic activity. Planta Med 1978;34:160-6. [Crossref] [PubMed]
- Vo NNQ, Fukushima EO, Muranaka T. Structure and hemolytic activity relationships of triterpenoid saponins and sapogenins. J Nat Med 2017;71:50-8. [Crossref] [PubMed]
- Ayatollahi H, Javan AO, Khajedaluee M, et al. Effect of Crocus sativus L. (saffron) on coagulation and anticoagulation systems in healthy volunteers. Phytother Res 2014;28:539-43. [Crossref] [PubMed]
- Farokhnia M, Shafiee Sabet M, Iranpour N, et al. Comparing the efficacy and safety of Crocus sativus L. with memantine in patients with moderate to severe Alzheimer's disease: a double-blind randomized clinical trial. Hum Psychopharmacol 2014;29:351-9. [Crossref] [PubMed]
- Mohamadpour AH, Ayati Z, Parizadeh MR, et al. Safety Evaluation of Crocin (a constituent of saffron) Tablets in Healthy Volunteers. Iran J Basic Med Sci 2013;16:39-46. [PubMed]
- Mufson EJ, Counts SE, Ginsberg SD, et al. Nerve Growth Factor Pathobiology During the Progression of Alzheimer’s Disease. Front Neurosci 2019; [Crossref] [PubMed]
- Fujii T, Yamada S, Misawa H, et al. Expression of Choline Acetyltransferase mRNA and Protein in T-Lymphocytes. Proc J Acad 1995;71 Ser. B:231-5.
- Dovey HF, John V, Anderson JP, et al. Functional gamma-secretase inhibitors reduce beta-amyloid peptide levels in brain. J Neurochem 2001;76:173-81. [Crossref] [PubMed]
- Cenini G, Voos W. Mitochondria as Potential Targets in Alzheimer Disease Therapy: An Update. Front Pharmacol 2019;10:902. [Crossref] [PubMed]
- Qin W, Yang T, Ho L, et al. Neuronal SIRT1 activation as a novel mechanism underlying the prevention of Alzheimer disease amyloid neuropathology by calorie restriction. J Biol Chem 2006;281:21745-54. [Crossref] [PubMed]
- Kim D, Nguyen MD, Dobbin MM, et al. SIRT1 deacetylase protects against neurodegeneration in models for Alzheimer’s disease and amyotrophic lateral sclerosis. EMBO J 2007;26:3169-79. [Crossref] [PubMed]
- Crowe MJ, Bresnahan JC, Shuman SL, et al. Apoptosis and delayed degeneration after spinal cord injury in rats and monkeys. Nat Med 1997;3:73-6. [Crossref] [PubMed]
- Hill IE, MacManus JP, Rasquinha I, et al. DNA fragmentation indicative of apoptosis following unilateral cerebral hypoxia-ischemia in the neonatal rat. Brain Res 1995;676:398-403. [Crossref] [PubMed]
- Pettmann B, Henderson CE. Neuronal cell death. Neuron 1998;20:633-47. [Crossref] [PubMed]
- Tanaka H, Fukuda N, Shoyama Y. Formation of monoclonal antibody against a major ginseng component, ginsenoside Rb1 and its characterization. Cytotechnology 1999;29:115-20. [Crossref] [PubMed]
- Fukuda N, Tanaka H, Shoyama Y. Formation of monoclonal antibody against a major ginseng component, ginsenoside Rg1 and its characterization. Cytotechnology 2000;34:197-204. [Crossref] [PubMed]
- Lu Z, Morinaga O, Tanaka H, et al. A quantitative ELISA using monoclonal antibody to survey paeoniflorin and albiflorin in crude drugs and traditional Chinese herbal medicines. Biol Pharm Bull 2003;26:862-6. [Crossref] [PubMed]
- Zhu SH, Shimokawa S, Tanaka H, et al. Development of an assay system for saikosaponin a using anti-saikosaponin a monoclonal antibodies. Biol Pharm Bull 2004;27:66-71. [Crossref] [PubMed]
- Shan S, Tanaka H, Shoyama Y. Enzyme-linked immunosorbent assay for glycyrrhizin using anti-glycyrrhizin monoclonal antibody and a new eastern blotting for glucronides of glycyrrhetinic acid. Anal Chem 2001;73:5784-90. [Crossref] [PubMed]
- Goto Y, Shima Y, Morimoto S, et al. Determination of tetrahydrocannabinolic acid-carrier protein conjugate by matrix-assisted laster desorption/Ionization mass spectrometry and antibody formation. Org Mass Spectr 1994;29:668-71. [Crossref]
- Xuan L, Tanaka H, Xu Y, et al. Preparation of monoclonal antibody against crocin and its characterization. Cytotechnology 1999;29:65-70. [Crossref] [PubMed]
- Sakata R, Shoyama Y, Murakami H. Production of monoclonal antibodies and enzyme immunoassy for typical adenylate cyclase activater, forskolin. Cytotechnology 1994;16:101-8. [Crossref] [PubMed]
- Ishiyama M, Shoyama Y, Murakami H, et al. Production of monoclonal antibodies and develp ment of an ELISA for solamargine. Cytotechnology 1995;18:153-8. [Crossref] [PubMed]
- Davis JM, Pennington JE, Kubler AM, et al. A simple, single-step technique for selecting and cloning hybridomas for the production of monoclonal antibodies. J Immunol Methods 1982;50:161-71. [Crossref] [PubMed]
- Mirshahi M, Amel Farzad S, Peyvandi M, et al. Evaluation of the osteogenic potential of crocin-incorporated collagen scaffold on the bone marrow mesenchymal stem cells. Drug Dev Ind Pharm 2021;47:1439-46. [Crossref] [PubMed]
- Sasamoto H, Suzuki S, Mardani-Korrani H, et al. Allelopathic activities of three carotenoids, neoxanthin, crocin and β-carotene, assayed using protoplast co-culture method with digital image analysis. Plant Biotechnol (Tokyo) 2021;38:101-7. [Crossref] [PubMed]
- Matsumoto M, Matsukawa N, Mineo H, et al. A Soluble Flavonoid-glycoside, αG-Rutin, Is Absorbed as Glycosides in the Isolated Gastric and Intestinal Mucosa. Biosci Biotechnol Biochem 2004;68:1929-34. [Crossref] [PubMed]
- Li J, Zhang S, Liu X, et al. Neuroprotective effects of leonurine against oxygen-glucose deprivation by targeting Cx36/CaMKII in PC12 cells. PLoS One 2018;13:e0200705. [Crossref] [PubMed]
- Ochiai T, Soeda S, Ohno S, et al. Crocin prevents the death of PC-12 cells through shpingomeylinase-ceramide signaling by increasing glutathione synthesis. Neurochem Int 2004;44:321-30. [Crossref] [PubMed]
- Charles I, Khalyfa A, Kumar DM, et al. Serum deprivation induces apoptotic cell death of transformed rat retinal ganglion cells via mitochondrial signaling pathways. Invest Ophthalmol Vis Sci 2005;46:1330-8. [Crossref] [PubMed]
- Caro-Maldonado A, Tait SW, Ramírez-Peinado S, et al. Glucose deprivation induces an atypical form of apoptosis mediated by caspase-8 in Bax-, Bak-deficient cells. Cell Death Differ 2010;17:1335-44. [Crossref] [PubMed]
- Soeda S, Ochiai T, Paopong L, et al. Crocin suppresses tumor necrosis factor-αinduced cell death of neuronally differentiated PC-12 cells. Life Sci 2001;69:2887-98. [Crossref] [PubMed]
- Oppenheim RW. Cell death during development of the nervous system. Annu Rev Neurosci 1991;14:453-501. [Crossref] [PubMed]
- Batistatou A, Green LA. Aurintricarboxylic acid rescues PC-12 cells and sympathetic neurons from cell death caused by nerve growth factor deprivation, correlation with suppression of endonuclease activity. J Cell Biol 1991;115:461-71. [Crossref] [PubMed]
- Rukenstein A, Rydel RE, Green LA. Multiple agents rescue PC-12 cells from serum-free cell death by translation and transcription-independent mechanisms. J Neurosci 1991;11:2552-63. [Crossref] [PubMed]
- Colombaioni L, Frago LM, Varela-Nieto I, et al. Serum deprivation increases ceramide levels and induces apoptosis in undifferentiated HN9.10e cells. Neurochem Int 2002;40:327-36. [Crossref] [PubMed]
- Verheij M, Bose R, Lin XH, et al. Requirement for seramide-initiated SAPF/JNK signaling in stress-induced apoptosis. Nature 1996;380:75-9. [Crossref] [PubMed]
- Asdaq SM, Inamdar MN. Potential of Crocus sativus (saffron) and its constituent, crocin, as hypolipidemic and antioxidant in rats. Appl Biochem Biotechnol 2010;162:358-72. [Crossref] [PubMed]
- Assimopoulou AN, Sinakos Z, Papageorgiou VP. Radical scavenging activity of Crocus sativus L. extract and its bioactive constituents. Phytother Res 2005;19:997-1000. [Crossref] [PubMed]
- Chen Y, Zhang H, Tian X, et al. Antioxidant potential of crocins and ethanol exracts of Gardenia jasminoides ELLIS and Crocus sativus L.: a relationship investigation between antioxidant activity and crocin content. Food Chem 2008;109:484-92. [Crossref]
- Ghadrdoost B, Vafaei AA, Rashidy-Pour A, et al. Protective effects of saffron extract and its active constituent crocin against oxidative stress and spatial learning and memory deficits induced by chronic stress in rats. Eur J Pharmacol 2011;667:222-9. [Crossref] [PubMed]
- Kanakis CD, Tarantilis PA, Tajmir-Riahi HA, et al. Crocetin, dimethylcrocetin, and safranal bind human serum albumin: stability and antioxidative properties. J Agric Food Chem 2007;55:970-7. [Crossref] [PubMed]
- Karimi E, Oskoueian E, Hendra R, et al. Evaluation of Crocus sativus L. stigma phenolic and flavonoid compounds and its antioxidant activity. Molecules 2010;15:6244-56. [Crossref] [PubMed]
- Makhlouf H, Saksouk M, Habib J, et al. Determination of antioxidant activity of saffron taken from the flower of Crocus sativus grown in Lebanon. Afr J Biotechnol 2011;10:8093-100. [Crossref]
- Pham TQ, Cormier F, Farnworth E, et al. Antioxidant properties of crocin from Gardenia jasminoides Ellis and study of the reactions of crocin with linoleic acid and crocin with oxygen. J Agric Food Chem 2000;48:1455-61. [Crossref] [PubMed]
- Sanchez-Vioque R, Rodrigues-Conde MF, Reina-Urena JV, et al. In vitro antioxidant and metal chelating properties of corm, petal and leaf from saffron (Crocus sativus L.). Ind Crop Prod 2012;39:149-53. [Crossref]
- Verma SK, Bordia A. Antioxidant property of Saffron in man. Indian J Med Sci 1998;52:205-7. [PubMed]
- Nakajima A, Yamada K, Zou LB, et al. Interleukin-6 protects PC12 cells from 4-hydroxynonenal-induced cytotoxicity by increasing intracellular glutathione levels. Free Radic Biol Med 2002;32:1324-32. [Crossref] [PubMed]
- Ban JY, Cho SO, Kwon SH, et al. Protection of Amyloid β Protein (25-35)-induced Neuronal Cell Damage by Methanol Extract of New Stem of Phyllostachys Nigra Munro Var. Henonis Stapf in Cultured Rat Cortical Neuron. Korean J Med Crop Sci 2005;13:95-102.
- Liu RT, Zou L, Lü Q. Liquiritigenin Inhibits Aβ25-35-Induced Neurotoxicity and Secretion of Aβ1-40 in Rat Hippocampal Neurons. Acta Pharmacol Sin 2009;30:899-906. [Crossref] [PubMed]
- Taheri R, Hadipour E, Tayarani-Najaran Z. Crocin protects against beta-amyloid peptide-induced apoptosis in PC-12 cells via the Pl3k pathway. Curr Mol Pharmacol 2021;14:627-34. [Crossref] [PubMed]
- Li J, Ding X, Zhang R, et al. Harpagoside ameliorates the amyloid-β-induced cognitive impairment in rats via up-regulating BDNF expression and MAPK/PI3K pathways. Neuroscience 2015;303:103-14. [Crossref] [PubMed]
- Adabizadeh M, Mehri S, Rajabpour M, et al. The effects of crocin on spatial memory impairment induced by hyoscine: Role of NMDA, AMPA, ERK, and CaMKII proteins in rat hippocampus. Iran J Basic Med Sci 2019;22:601-9. [PubMed]
- Lisman JE, Goldring MA. Feasibility of long-term storage of graded information by the Ca+±independent form of Ca2+/calmodulin-dependent protein kinase molecules of the postsynapic density. Proc Natl Acad Sci USA 1988;85:5320-4. [Crossref] [PubMed]
- Silva AJ, Stevens CF, Tonegawa S, et al. Deficient hippocampal long-term potentiation in alpha-calcim-calmodulin kinase II mutant mice. Science 1992;257:201-6. [Crossref] [PubMed]
- Kury S, van Wperden GM, Besnard T, et al. Undiagnoused diseases network; GEM HUGO; deciphering developmental disorders study. De novo mutatons in proteins kinase genes CaMK2A and Camk2b cause neurodevelopmental disorders. Ann Clin Transl Neurol 2018;5:280-96.
- Chang JY, Patta-Bueno P, Laviv T, et al. CaMKII autophosphorylation is necessary for optimal integration of Ca++ signal during LTP induction, but not maintenance. Neuron 2017;94:800-8.e4. [PubMed]
- Radak D, Katsiki N, Resanovic I, et al. Apoptosis and Acute Brain Ischemia in Ischemic Stroke. Curr Vasc Pharmacol 2017;15:115-22. [Crossref] [PubMed]
Cite this article as: Soeda S, Ohta T, Uto T, Shoyama Y. The effect of crocin on neuroprotective activity in vitro: a narrative review. Longhua Chin Med 2022;5:24.