Neuroprotective activities of icariin in rodent models of Alzheimer’s disease: a systematic review and meta-analysis
Introduction
Alzheimer’s disease (AD) is a neurodegenerative disease, which is irreversible and considered to be the most common cause of dementia, accounting for 50–70% of dementia patients worldwide (1). The clinical symptoms of AD are mainly short-term memory loss, spatial orientation disorders, and personality and behavior changes. In 2020, the official website of the World Health Organization (WHO) published that there were about 50 million people with dementia all over the world, and the number of patients is expected to rise to 152 million in 30 years.
The pathogenesis of AD is complex, which is generally thought to be related to environmental and genetic factors. The typical pathological features are the deposition of amyloid beta (Aβ) plaques, the accumulation of neurofibrillary tangles (NFTs), and the death of neurons accompanied by loss of synapses (2). At present, a variety of hypotheses have been proposed to clarify the pathogenesis of AD, among which the most widely accepted hypotheses cite the Aβ-cascade, tau hyperphosphorylation, oxidative stress, inflammatory response, and metabolic disorders (3,4). Despite much research, the commonly used therapeutic drugs, such as donepezil and memantine, can only alleviate the symptoms of AD patients, but cannot reverse or prevent the development of the disease, and also have certain side effects. Therefore, research and development of new effective drugs is a hot topic in AD research.
Icariin is a major flavonoid component extracted from the Chinese herb Epimedium brevicornum Maxim. (Berberidaceae; name in English, Shorthorn Barrenwort; name in Chinese, Yinyanghuo). Pharmacological studies have shown that icariin has protective effects on the cardiovascular system, as well as immunoregulatory, anti-oxidant, anti-aging and anti-inflammatory properties (5-7). In recent years, many studies have found that icariin also has significant neuroprotective effects. For example, in APP transgenic mice, icariin alleviated the impairment of learning and memory by decreasing the expressions of APP and β-secretase expression (BACE1) (8). In APP/PS1 mice, icariin improved cognitive function by inhibiting the endoplasmic reticulum (ER) stress signal pathway (9).
There have been many studies to evaluate icariin in AD models. No single study can provide sufficient, comprehensive information on the efficacy and mechanism of icariin in the treatment of AD, yet there has been no review of these studies to summarize and systematically evaluate their findings. Therefore, this review took a scientific approach to eliminate bias against each study, comprehensively evaluated the effects of icariin in different rodent models of AD, and summarized the neuroprotective mechanisms of icariin. It is hoped that this summary evaluation can provide reliable evidence and insight for developing icariin into a clinical drug.
We present the following article in accordance with the PRISMA reporting checklist (available at https://lcm.amegroups.com/article/view/10.21037/lcm-21-50/rc).
Methods
Search strategy
To study the neuroprotective effect of icariin in rodent models of AD, we carefully searched the literature in three independent literature databases, PubMed, Web of Science and Google Scholar from inception to July 2021. The keywords of the search were “icariin (Title/Abstract)” and “Alzheimer’s disease (Title/Abstract)” OR “Alzheimer disease (Title/Abstract)”. The study was limited to preclinical studies in rodent models. The reviewers (Yu-Xuan Kan and Cheng-Fu Su) screened articles independently by reading the title and full-text according to eligibility criteria.
Eligibility criteria
Inclusion criteria
- Study on evaluating the effect of icariin on AD in vivo;
- AD rodent models of any gender, age, or weight;
- Any dosage, treatment time and mode of administration;
- Inclusion of at least one icariin treatment group and a control group with placebo.
Exclusion criteria
- Repetitive studies, review articles, articles without access rights;
- In vitro studies;
- Icariin was used in combination with other drugs;
- Failure to evaluate the effects of icariin on AD rodent models.
Data extraction
Two investigators separately screened the studies and performed data collection procedures. Disputes during the process were resolved after discussion by a third party. According to the inclusion criteria, the basic information of the selected studies is as follows (Table 1): (I) authors and publication year; (II) animal information, including gender, age, species, weight and modeling methods; (III) icariin treatment, including administration methods, dosage and duration time; (IV) evaluation indicators; (V) pharmacological activity/mechanisms. Image J software was used to extract the data of the result graph.
Table 1
Authors/year | Model | Icariin intervention | Evaluation indicators | Pharmacological activity/mechanisms |
---|---|---|---|---|
Zhang et al./2018 (10) | APP/PS1 transgenic mice (C57BL/6J, male, 6 months old, 28.8±5 g) | Icariin (120 mg/kg/d); orally administered for 3 months | Flame atomic absorption spectroscopy (iron level); ELISA (IL-1β, IL-6, TNF-α levels); colorimetry (GSH-Px, SOD, MDA activities) | Suppress iron overload (anti-inflammatory, anti-oxidant) |
Li et al./2019 (11) | APP/PS1 transgenic mice (C57BL/6J, male, 5 months old) | Icariin (100 mg/kg/d) orally administered for 10 days | Behavioral tests (nest-building assay, social interaction assay); IHC (Aβ deposits, plaque numbers) | Restored behavioral function, ameliorated Aβ deposits in both the cortex and hippocampus |
Jiang et al./2019 (12) | SD rats (3–4 months old, 250±50 g) injected with Aβ1–42 | Icariin (30, 60 and 90 mg/d) orally administered for 4 weeks | Behavioral tests (MWM); ELISA (Aβ42 level); biochemical estimations (Cathepsin D, MDA, SOD activities); WB (APP, 2211, LC3, Beclin 1, AKT, pS473AKT, etc.); IHC (cleaved-caspase-3, 6E10, Beclin 1, GFAP); TEM; Nissl staining | Improved cognitive deficits, reduced Aβ deposits (reverses autophagiclysosomal dysfunction, phosphorylation of p70↑); Anti-apoptosis (cleaved-caspase-3↓, phosphorylation of AKT↑) |
Zhang et al./2014 (13) | APP/PS1 transgenic mice (C57BL/6J, male & female, 5 months old) | Icariin (100 mg/kg/d) orally administered for 10 days | Behavioral tests (nesting assay); IHC (Aβ, IBA-1, GFAP) | Improved nesting capacity, reduced Aβ deposits (anti-inflammatory, TGF-β1↑) |
Wang et al./2019 (14) | APP/PS1 transgenic mice (male, 3 months old, 24–30 g) | Icariin (60 mg/kg/d) orally administered for 6 months | Behavioral tests (open field test, elevated plus maze, Y maze, MWM); sucrose preference test; ELISA (IL-1β, IL-6, TNF-α, IL-4, IL-10, TGF-β1, NF-κB p65); WB (PPARγ); IHC (Aβ1–42, Iba-1 and iNOS) | Improved spatial memory impairment, inhibited Aβ accumulation and M1 activation of microglia (PPARγ↑) |
Sheng et al./2017 (15) | SD rats (male, 200–250 g) injected with Aβ1–42 | Icariin (30, 60 and 120 mg/kg/d) orally administered for 28 days | Behavioral tests (MWM); TEM (synaptic ultrastructure); IHC (BDNF and pCREB); WB and qPCR (PSD-95, BDNF, pTrkB, TrkB, pAKT, AKT, CREB, pCREB) | Alleviated cognitive impairment, improved synaptic plasticity (regulated BDNF/TrkB/Akt pathway) |
Li et al./2015 (16) | Tg 2576 mice (male & female, 9 months old) | Icariin (60 mg/kg/d) orally administered for 3 months | Behavioral tests (Y maze test); IF (BrdU and NeuN); ELISA (Aβ1–40 and Aβ1–42 levels); WB (APP) | Improved spatial working memory (APP, insoluble Aβ↓) |
Zhang et al./2014 (8) | APPV717I transgenic mice (male & female, 4 months old) | Icariin (30 and 100 μmol/kg/d) orally administered for 6 months | Behavioral tests (MWM, object recognition test); WB, IHC and ELISA (Aβ, APP, BACE-1, PS1) | Improved learning-memory abilities, reduced Aβ plaque deposition (APP, BACE-1↑) |
Chen et al./2019 (17) | SAMP8 mice (male, 5 months old) | Icariin (20, 40 and 80 mg/kg/d) orally administered for 3 months | Behavioral tests (MWM); SA-β-gal staining (SA-β-gal activity); Nissl staining; TEM (the hippocampal neuronal ultrastructure); WB (LC3-II, p62) | Delay brain aging (regulated autophagy) |
Wu et al./2020 (18) | SAMP8 mice (male, 19–23 g, 6 months old) | Icariin (60 mg/kg/d) orally administered for 22 days | Behavioral tests (MWM); HE staining (histopathological changes); IHC (Bax, Bcl-2); WB (Aβ1–42, BACE1) | Improve cognitive function (reduced Aβ deposition, anti-apoptosis) |
Urano et al./2010 (19) | 5xFAD transgenic mice (female, 7–9 months old) | Icariin (50 μmol/kg/d) orally administered for 8 days | Behavioral tests (MWM, open field test) | Improved spatial memory impairment |
Li et al./2019 (9) | APP/PS1 transgenic mice (male, 9 months old, 35–45 g) | Icariin (60 mg/kg/d) orally administered for 3 months | Behavioral tests (MWM, object recognition test); Nissl staining; IF (Aβ deposition); TUNEL staining; qPCR (TRB3, GADD34, ERO1α); WB (APP, Aβ1–42, Aβ1–40, BACE1, ADAM10, GRP78, ATF6, PERK, eIF2α, ATF4, CHOP, caspase-3, etc.) | Suppressed ER stress (inhibiting PERK/eIF2α pathway) |
Zhang et al./2015 (20) | APPV717I transgenic mice (male & female, 4 months old) | Icariin (30 and 100 μmol/kg/d) orally administered for 6 months | WB (α-synuclein, Parkin, UCH-L1, HSP70); IHC and PCR (α-synuclein) | Inhibited the overexpression of α-synuclein (Parkin, UCH-L1, HSP70↑) |
Zong et al./2016 (21) | SD rats (male, 3 months old, 220–250 g) induced with IBO | Icariin (10, 20 and 40 mg/kg/d) orally administered for 20 days | Behavioral tests (MWM); HE staining, Nissl staining and TUNEL assay; WB (Bax, Bcl-2, pro-caspase-3, MAPK family, NF-κB, calbindin-D28k) | Anti-excitotoxicity (inhibited MAPK and NF-κB pathway), regulated Ca2+ homeostasis (calbindin-D28) |
Li et al./2015 (22) | SD rats (male, 10 weeks old, 250±20 g) with ligation of the bilateral common carotid arteries | Icariin (10 and 40 mg/kg/d) orally administered for 23 days | Behavioral tests (MWM); HE staining; WB (Aβ1–40, Aβ1–42, APP, BACE1, ADAM10, IDE, Smad2/3, TGF-β1) | Alleviated cognitive deficits, reduced Aβ deposition (APP↓, BACE1↓, ADAM10↑, IDE↑, TGF-β1↓, p-Smad2/3↓) |
Jin et al./2014 (23) | APP/PS1 transgenic mice (male, 10 months old) | Icariin (30 and 60 mg/kg/d) orally administered for 4 months | Behavioral tests (Y maze); qPCR and WB (APP, nNOS, iNOS, eNOS, Aβ1–40, Aβ1–42); ELISA (PDE5, cGMP); colorimetry (NO production) | Improved learning and memory abilities (PDE5↓, activated NO/cGMP signal pathway) |
Zhu et al./2019 (24) | APP/PS1 transgenic mice (male, 4 months old) | Icariin (60 mg/kg/d) orally administered for 8 months | Behavioral tests (MWM); ELISA (Aβ40 and Aβ42 levels); flow cytometry (the ratio of CD4+ and CD8+ T cells); cytokine array analysis (IL-1α, IL-1β, IL-6, IL-10, IL-12p70, IL-17A, MCP-1, IFN-γ, TNF-α, and GM-CSF etc.) | Alleviated cognitive deficits, reduce Aβ deposition, anti-inflammation (pro-inflammatory cytokines ↑, modulated CD4+ T cell differentiation) |
Chen et al./2016 (25) | 3xTg-AD mice (female, 2 months old) | Icariin (65 mg/kg/d) orally administered for 6 months | Behavioral tests (MWM, place navigation test, probe trial test); magnetic resonance spectroscopy (brain metabolites); WB (PDH1a, COX IV, PSD95, BACE1, synaptophysin); ELISA (Aβ1–42 level); biochemical estimations (ATP level) | Improved spatial learning and memory deficits, reduce Aβ plaque deposition (BACE1↓), modulated brain metabolites, improved mitochondrial function (PDH1a, COX IV, PSD95, synaptophysin↑) |
Nie et al./2010 (26) | Wistar rats (male, 6 months old, 400–600 g) induced with Aβ25–35 | Icariin (30, 60 and 120 mg/kg/d) orally administered for 14 days | Behavioral tests (MWM); IHC (APP, Aβ1–40); qPCR (β-secretase, SOD-2) | Improved learning and memory abilities, reduced Aβ deposition (β-secretase↓, SOD2↑) |
“↑” means up-regulation, “↓” means down-regulation. IHC, immunohistochemistry; Aβ, amyloid beta; SD, Sprague-Dawley; MWM, Morris water maze; WB, western blot; TEM, transmission electron microscopy; qPCR, quantitative real-time polymerase chain reaction; SAMP8, senescence-accelerated mouse prone 8; HE, hematoxylin and eosin; IF, immunofluorescence; ER, endoplasmic reticulum; IBO, ibotenic acid.
Quality assessment
According to the characteristics of AD experimental study, the methodological quality assessment form was established by adjusting the basis of the CAMARADES study quality checklist (27,28). The assessment criteria included: (I) peer-reviewed publication; (II) sample size calculation; (III) random allocation sequence; (IV) appropriate animal models of AD; (V) blinded intervention; (VI) blinded evaluation of outcome; (VII) compliance with animal welfare regulations; (VIII) statement of conflict of interest. Each study had a quality score of no more than 7 points. “Y”, “N”, and “U” were used to indicate low, high and uncertain risk of bias, respectively.
Statistical analysis
RevMan 5.3 software were used for statistical analysis. Each outcome was continuous data, represented by mean difference (MD) and 95% confidence interval (CI). Heterogeneity in included studies was evaluated by I-square (I2) statistical tests. I2>50 was considered that there is significantly heterogeneity.
Results
Study selection
Preliminary searches of the data resources were carried out according to the above method, and a total of 139 articles were identified (114 articles from Web of Science and PubMed databases, 25 articles from Google Scholar). By browsing titles and abstracts, we selected 25 articles on the effects of icariin on AD. After reading the full text, we determined that, 19 of these articles qualified for meta-analysis (Figure 1).
Of the 19 articles selected, 14 studies chose mice and 5 studies chose rats. Among the studies on the effects of icariin on mice models, 8 studies used male mice, 2 studies used female mice, and 4 studies used both male and female. In the rat models, 4 studies used male rats and the other one study did not mention gender.
Study characteristics
Different animal models
In this review, five kinds of rodent models were used in the 19 studies. They were: Aβ-induced and ibotenic acid (IBO)-induced animal models; APP/PS1/tau transgenic models; senescence-accelerated mouse prone 8 (SAMP8) model; and the permanent bilateral common carotid artery occlusion (BCCAO) model. We summarized the different animal models in Table 2.
Table 2
Model | Mechanism | Main uses of the model | Disadvantages |
---|---|---|---|
Transgenic mice | Aβ accumulation and NFT formation in the brain | Studying effects of APP, PS1 or tau mutations in the pathogenesis of AD | Lack of changes of APP and tau metabolism |
SAMP8 | A rapidly aging mouse model with cognitive impairment | Studying age-related spatial learning and memory impairment of AD | Expensive, weak reproduction |
Aβ-induced | Neurotoxicity of Aβ peptide | Studying deposition, acute toxic of Aβ in AD | As an acute model, inconsistent with the pathological changes of slow Aβ deposition in the brains of AD patients |
IBO-induced | Excitatory toxicity leads to basal ganglia damage | Studying neuron apoptosis and mild cognitive impairment of AD | Cannot reflect the etiology of AD |
BCCAO | Chronic cerebral ischemia and hypoxia lead to impaired brain function | Studying cognitive dysfunction associated with chronic cerebral hypoperfusion in AD | Complex surgical procedure, low survival rate, short life spans |
AD, Alzheimer’s disease; SAMP8, senescence-accelerated mouse prone 8; Aβ, amyloid beta; IBO, ibotenic acid; BCCAO, bilateral common carotid artery occlusion; NFT, neurofibrillary tangle.
The transgenic mice model is an ideal tool to study the pathogenesis of AD. Currently, Tg 2576, APPV717I, APP/PS1, 3xTg-AD, and 5xFAD transgenic mice have been widely used in experimental studies. Tg 2576 mice, the first transgenic mice model of AD showing cognitive impairment, express the 695 aa long isoform of human APP containing the K670N/671L double mutation. They have amyloid deposits in the cortex and limbic areas of the brain at 9 months, along with behavioral deficits (16,29).The APPV717I transgenic mice carry the V717I mutation, resulting in the increased level of Aβ42 and AD-like pathological features (30,31).The APP/PS1 mice model has Aβ deposition and neurocognitive impairment due to APP and PS1 overexpression. Aβ can be detected in this model at 6 months old, and after 3 months, extracellular Aβ deposition is evident in the cortex, accompanied by significant impairments in synaptic transmission and long-term potentiation (32,33).3xTg-AD mice harboring the PS1M146V, APPSwe, and tauP301L transgenes mimic both amyloid and tau AD neuropathologies. Extracellular Aβ deposition is increased significantly and mainly distributed in the hippocampus and frontal cortex (34).The 5xFAD transgenic mice carry five mutated neuron-specific genes, including Swedish (K670N and M671L), Florida (I716V) and London (V717I) mutations in APP, and M146L and L286V mutations in PS1. They have similar pathological characteristics to AD, especially the aggregation of Aβ in neurons at 1.5 months of age, indicating that this model is particularly relevant for the study of the early stage of AD (19,32,35).
Besides the transgenic mice models mentioned above, chemical-induced AD models such as IBO-induced and Aβ-induced are also used in AD studies. IBO is a neurotoxin, which can cause neuron degeneration, apoptosis, and mild cognitive impairment. Injecting IBO into the brains of animals, such as monkeys and rats, can cause pathological features similar to human AD patients (36,37). Aβ deposition is an important pathological mechanism of AD. The Aβ-induced model is generally established by multiple injections of Aβ fragments into the hippocampal CA1 area or lateral ventricle. It is considered to be a non-genetic model of AD. Many studies have shown that injection of Aβ can cause Aβ deposition, tau hyperphosphorylation, Ca2+ influx, ROS production (38). Excessive deposition of Aβ may lead to high production of senile plaques and NFTs, while hyperphosphorylated tau and Aβ can further exacerbate neurotoxicity and lead to dementia (39,40).
In addition to the transgenic mice models and chemical-induced models, SAMP8 mice and BCCAO model are also used. The SAMP8 mice model is a spontaneous animal model of accelerated senescence. These mice are characterized by hyperphosphorylation of tau-like NFTs with age, and they develop learning and memory deficits at about 8 months of age (41-43). The decrease of cerebral blood flow is another important factor of cognitive dysfunction, which is common in the elderly (44,45). Previous studies have shown that the BCCAO model induces cognitive impairment and neuron loss (46,47). Therefore, this model is considered to be a chronic cerebral perfusion model that can better simulate the pathological development of human AD (48).
Behavioral test analysis
Behavioral test analysis is an important research method in AD research. By observing the behavior of experimental animals in a specific environment, we can analyze the neurological changes in mice. Morris water maze (MWM) was used in most of the included studies. Also, Y maze, open field test, and object recognition test were used in some of the studies.
MWM was proposed and applied by the British psychologist Morris in the 1980s (49). It is an important detection method for analyzing the spatial sense and learning ability of experimental animals. By comparing and analyzing the changes in spatial cognition, learning and memory of AD model animals treated or untreated with drugs, we can evaluate the effectiveness of the intervention, in this case, icariin, to alleviate AD (50). In a typical MWM, escape latency of experimental animals is recorded during the training period, and then a probe test is performed by removing the platform. For each animal, the time spent in the target quadrant and the number of times crossing the platform are recorded. The same test is performed again after administration of icariin. According to the changes in the indexes of each group, the effect of icariin on cognitive function of the AD animals was evaluated.
In this review, 13 of the 19 studies performed the MWM test, and only 5 of them recorded the number of times crossing the platform. A total of 52 animals (SAMP8, n=10; Aβ-induced rats, n=12; transgenic mice, n=30) were enrolled and given different doses of icariin, while 51 vehicle-treated animals were added as controls. Image J and RevMan 5.3 software were used to extract and analyze the number of times crossing the platform in these five studies. The results are shown in the forest plot in Figure 2. Compared with vehicle-treated animals, icariin significantly increased the number of times crossing the platform in these three different AD animal models (MD =2.41, 1.72 and 1.28). Furthermore, there was no significant heterogeneity among the subgroups of transgenic mice (P=0.20; I2=39%). The analysis of combined subgroups showed that icariin treatment significantly improved cognitive function in AD rodent models (MD =1.70; 95% CI: 1.46 to 1.94; P<0.00001), with significant heterogeneity (P=0.0007; I2=79%). The reason for the elevated heterogeneity may be related to different AD animal models.
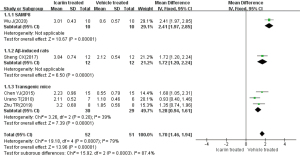
Neuroprotective mechanisms analysis
We summarized the neuroprotective mechanisms of icariin in the included studies (Figure 3). There are four main mechanisms, namely, reduction of Aβ deposition, anti-inflammation, anti-oxidant activity, and regulation of autophagy. Furthermore, some studies reported that icariin inhibited apoptosis and regulated synaptic plasticity.
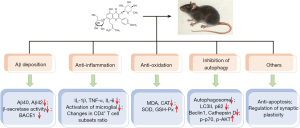
Thirteen studies demonstrated that icariin inhibited the increase of Aβ levels in AD rodent models. Li et al. (11) reported that treatment with icariin decreased the number of plaques (P<0.05) and Aβ immune-reactivity areas (P<0.05) in the hippocampus and cortex compared with the control group. Jiang et al. (12) found that the level of Aβ42 declined in icariin-treated AD model rats (P<0.01). They performed immunohistochemical studies using a 6E10 antibody bound to amino acids 1–16 of Aβ to assess the distribution of Aβ clearly. The results showed that icariin reduced 6E10 positive staining (P<0.05). Zhang et al. (13) and Wang et al. (14) demonstrated that icariin administration decreased the number of Aβ plaques in AD models. Li et al. (9) showed that treatment with icariin reduced the Aβ burden (P<0.01) and increased the expression of neuroprotective ADAM10 protein (P<0.01) in the hippocampus of APP/PS1 mice. Li et al. (22) reported that, in BCCAO rats, icariin administration decreased the level of Aβ through multiple targets, such as by reducing the production of Aβ and by increasing the degradation of Aβ, which may be related to the TGFβ1 signaling pathway. Jin et al. (23) showed a significant decrease in the levels of Aβ and PDE5 mRNA or protein in icariin-treated transgenic mice. Li et al. (16) and Zhu et al. (24) reported that icariin lowered the insoluble Aβ40 and Aβ42 levels in the brain, but had no significant effect on soluble Aβ. Nie et al. (26) showed that icariin inhibited Aβ1–40 and mRNA levels of β-secretase in Aβ25–35-induced rats in a dose-dependent manner. They hypothesized that icariin may reduce the production of Aβ peptide by inhibiting β-secretase, thus improving the cognitive function in AD rats. Using APP transgenic mice or SAMP8 mice, Zhang et al. (8), Wu et al. (18) and Chen et al. (25) all found that icariin alleviated Aβ deposition by decreasing the expression of BACE1, thus improving cognitive function.
Four of the included studies assessed the level of Aβ in the brains of rodent animals by ELISA. A total of 33 rodent animals (mice and rats, n=23 and n=10) were treated with different doses of icariin, while 33 vehicle-treated animals were added as controls (mice and rats, n=23 and n=10). The level of Aβ was analyzed using a forest plot in RevMan 5.3 software (Figure 4). After icariin treatment, Aβ in the brain was significantly reduced compared with the model group (MD =−2.75; 95% CI: −3.29 to −2.22; P<0.00001). There was high heterogeneity among the subgroups (I2=99%), which may be related to the different species of experimental animals, modeling methods and detection methods, as well as differences in the dose and time of icariin administration.
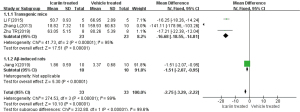
In recent years, more attention has been paid to the role of neuroinflammation in AD. Microglia appear to be important. Activated microglia can be divided into M1 and M2 phenotypes. M1 phenotype releases a large number of pro-inflammatory cytokines to cause neurotoxicity, while M2 phenotype releases mediators such as IL-4 and IL-1 to inhibit the inflammatory response (51). Therefore, inhibiting the activation of microglia and the massive production of inflammatory cytokines may be an effective strategy to alleviate AD. Four studies have indicated that icariin has an anti-inflammatory effect. Zhang et al. (10) showed that the levels of TNF-α, IL-1 and IL-6 were decreased in icariin-treated transgenic mice compared with the model group (P<0.05). Zhang et al. (13) reported that icariin inhibited the activation of microglial (P<0.05) and the level of TGF-β1 (P<0.05) in the hippocampus and cortex, and alleviated behavioral deficits. Studies confirmed that PPAR-γ can attenuate M1 activation of microglia and mediate its transformation to the M2 phenotype (52,53). Wang et al. (14) found that treatment with icariin decreased the levels of IL-1β, TNF-α and IL-6, and increased the levels of IL-4, IL-10 and TGF-β in the hippocampus and prefrontal cortex of APP/PS1 mice (P<0.05). These changes may be related to PPAR-γ inhibiting the activation of M1 microglia. Zhu et al. (24) reported that icariin regulated the differentiation of CD4+ T cells and inhibited the release of pro-inflammatory cytokines in plasma and brain tissue.
Oxidative stress is one of the earliest events in the pathological development of AD. When the body is stimulated by certain conditions, excessive oxygen free radicals are produced, which greatly exceed the capacity of the anti-oxidant system, resulting in oxidative stress and neuron damage. Superoxide dismutase (SOD) and glutathione peroxidase (GSH), which can remove excessive free radicals, are the first line of defense against oxidation in the body. Malondialdehyde (MDA) is the product of lipid peroxidation, which indirectly reflects the level of oxidative stress. Three studies have shown the anti-oxidant function of icariin in AD rodent models. Zhang et al. (10) reported that icariin decreased MDA (P<0.01) and increased the activities of SOD (P<0.01) and GSH (P<0.01) in the brains of APP/PS1 transgenic mice, thus alleviating the tissue damage caused by iron overload. Nie et al. (26) and Jiang et al. (12) demonstrated that treatment with icariin inhibited oxidative stress by increasing SOD-2 mRNA expression (P<0.05) or regulating SOD (P<0.05) and MDA (P<0.05) levels in Aβ-induced AD rat models respectively.
Two studies indicated that the alleviation of icariin on cognitive impairment is related to autophagy. Jiang et al. (12) reported that treatment with icariin reduced the levels of LC3II (P<0.01), Beclin1(P<0.01) and Cathepsin D (P<0.05), and increased the phosphorylation of p70S6K (P<0.01) in Aβ-induced rats, suggesting that it reversed Aβ-induced autophagy activation. Meanwhile, icariin inhibited apoptosis by increasing the ratio of pS473AKT/AKT. Chen et al. (17) showed that icariin administration reduced the number of autophagosomes in hippocampal neurons of SAMP8 mice, and inhibited the expressions of LC3-II and p62 in the hippocampus and cortex (P<0.05), suggesting that the cognitive function of SAMP8 mice could be improved by regulating autophagy.
The neuroprotective mechanisms of icariin also include anti-apoptosis and regulation of synaptic plasticity. Wu et al. (18) reported that icariin treatment increased the level of anti-apoptotic protein Bcl-2 and reduced the level of pro-apoptotic protein Bax, thus inhibiting neuronal apoptosis. Sheng et al. (15) found that icariin restored normal synaptic morphology and increased the expression of postsynaptic density protein 95 (PSD95), which helped improve cognitive function in AD mice.
Methodological quality
In assessing the methodological quality of studies, it is necessary to evaluate the various risks of bias that may arise in the design, implementation, and result analysis of individual studies. According to the characteristics of AD experimental study, the methodological quality assessment form was established by adjusting the basis of the CAMARADES study quality checklist. The results were shown in Table 3, the methodological quality of each study ranged from 3 to 5 (highest possible score, 8). All included studies were published in peer-reviewed journals. Random allocation sequence was reported by 17 publications (89.5%). Compliance with animal welfare regulations and conflict of interest were mentioned in 18 publications (94.7%) and 16 publications (84%), respectively. All studies failed to mention whether blind intervention and blind evaluation of outcome were used, and no study performed sample size calculation. Appropriate sample size is important for animal experiments, and the number of animals selected should be sufficient for statistical analysis while avoiding unnecessary sacrifices.
Table 3
Authors/year | 1 | 2 | 3 | 4 | 5 | 6 | 7 | 8 | Total |
---|---|---|---|---|---|---|---|---|---|
Zhang et al./2018 (10) | Y | N | Y | Y | U | U | Y | Y | 5 |
Li et al./2019 (11) | Y | N | N | Y | U | U | Y | Y | 4 |
Jiang et al./2019 (12) | Y | N | Y | Y | U | U | Y | Y | 5 |
Zhang et al./2014 (13) | Y | N | Y | Y | U | U | Y | N | 4 |
Wang et al./2019 (14) | Y | N | Y | Y | U | U | Y | N | 4 |
Sheng et al./2017 (15) | Y | N | Y | Y | U | U | Y | Y | 5 |
Li et al./2015 (16) | Y | N | Y | Y | U | U | Y | Y | 5 |
Zhang et al./2014 (8) | Y | N | Y | Y | U | U | Y | Y | 5 |
Chen et al./2019 (17) | Y | N | Y | Y | U | U | Y | N | 4 |
Wu et al./2020 (18) | Y | N | Y | Y | U | U | Y | Y | 5 |
Urano et al./2010 (19) | Y | N | N | Y | U | U | Y | Y | 4 |
Li et al./2019 (9) | Y | N | Y | Y | U | U | Y | Y | 5 |
Zhang et al./2015 (20) | Y | N | Y | Y | U | U | Y | Y | 5 |
Zong et al./2016 (21) | Y | N | Y | Y | U | U | Y | Y | 5 |
Li et al./2015 (22) | Y | N | Y | Y | U | U | Y | Y | 5 |
Jin et al./2014 (23) | Y | N | Y | Y | U | U | Y | Y | 5 |
Zhu et al./2019 (24) | Y | N | Y | Y | U | U | Y | Y | 5 |
Chen et al./2015 (25) | Y | N | Y | Y | U | U | Y | Y | 5 |
Nie et al./2010 (26) | Y | N | Y | Y | U | U | N | N | 3 |
1: peer reviewed publication; 2: sample size calculation; 3: random allocation sequence; 4: appropriate animal models of AD; 5: blinded intervention; 6: blinded evaluation of outcome; 7: compliance with animal welfare regulations; 8: statement of conflict of interest; Y: yes; N: no; U: unclear. AD, Alzheimer’s disease.
Discussion
In recent years, many studies have focused on the treatment of neurodegenerative diseases with Chinese herbal extracts. Icariin is a flavonoid in Epimedium, a traditional Chinese herbal medicine. Many studies have shown that icariin has neuroprotective effects and can improve cognitive function in AD animal models (14,15). Meta-analysis is used to evaluate multiple trials of a single intervention because it can eliminate bias in individual studies. This is of great significance for evaluating the efficacy of drugs in preclinical trials. This review used meta-analysis to systematically evaluate the neuroprotective effects of icariin in different rodent models of AD, providing some reference for future clinical studies.
In this review, 19 studies of icariin in rodent models of AD were selected from three databases and systematically evaluated. Among them, 13 studies all used the MWM test to assess the effects of icariin on learning and spatial memory abilities in AD animals. This review conducted a meta-analysis on the number of times crossing the platform by forest plot. The results showed that icariin treatment significantly increased crossing times in animals compared with the control groups, suggesting that icariin improves cognitive function in AD rodent models.
A dependable animal model plays an essential role in exploring the pathogenesis and drug development of AD. In the included studies, 12 studies used AD transgenic mice, 3 studies used Aβ-induced AD models, 2 studies used SAMP8 mice, one study used an IBO-induced model, and one study used a BCCAO model. Different models have their own characteristics. For example, the Aβ-induced model is an acute model, which may cause some damage to the brain tissue of animals during the modeling process. This model is inconsistent with the pathological changes of slow Aβ deposition in the brains of human AD patients. SAMP8 mice are considered as a model of sporadic AD. Since over 95% of human AD patients are sporadic, it could be argued that these mice are a much more relevant pre-clinical model. But it is relatively expensive. Meanwhile, transgenic mice also commonly used AD animal model at present. They carry multiple AD-related pathogenic genes and can more fully simulate the pathological characteristics of Aβ deposition, plaque formation and neuron loss in the brain of AD patients.
In this review, the neuroprotective mechanisms of icariin are systematically introduced, mainly include reducing Aβ deposition, anti-inflammation, anti-oxidation and autophagy regulation. In 13 studies using transgenic mice, the neuroprotective mechanisms of icariin mainly concentrate on anti-Aβ deposition (4 studies) and anti-inflammation (4 studies). In addition, one study showed that icariin played a neuroprotective role by inhibiting neurons apoptosis, and one study showed that icariin regulated synaptic plasticity through BDNF/TrkB/Akt pathway.
This review also has some limitations. By assessing the methodological quality of each study for the various risks of bias that may occur during the design, implementation, and analysis of results, we found that: The quality of the studies is inconsistent and there is a general lack of high-quality evidence. All studies lacked the calculation of sample size. The number of animals in each group may affect the significance of the differences between groups. In addition, we did not conduct subgroup analysis of icariin dosage, so the effect of dosage on the efficacy of icariin needs further study.
In conclusion, we systematically reviewed 19 studies of the effects of icariin on AD rodent models, and summarized the mechanisms. Icariin has a significant neuroprotective effect on different AD models, mainly through reducing Aβ deposition, anti-inflammation, anti-oxidation and regulating autophagy. The results of this review will provide useful information for the design of future experiments related to the neuroprotective effects of icariin and provide a basis for the further development of icariin as an anti-AD drug.
Acknowledgments
We would like to thank Dr. Martha Dahlen for her English editing of this manuscript.
Funding: This work was supported by the Health and Medical Research Fund (HMRF/17182541, HMRF/17182551) from the Food and Health Bureau of Hong Kong Government and the General Research Fund (HKBU 12101417, HKBU12100618) from the University Grants Committee of Hong Kong Government. The work was partly supported by the Research Fund from Hong Kong Baptist University (RC-IRCs/17-18/03, IRCMS/19-20/H02), and also supported by the National Natural Science Foundation of China (NSFC 81703487, NSFC 81773926) and Basic Research Fund from Shenzhen Science and Technology Program (JCYJ20180507184656626, JCYJ20180302174028790).
Footnote
Provenance and Peer Review: This article was commissioned by the Guest Editors (Zhi-Xiu Lin, Yanfang Xian and Hongxi Xu) for the series “Pharmacology of Chinese Herbal Medicine” published in Longhua Chinese Medicine. The article has undergone external peer review.
Reporting Checklist: The authors have completed the PRISMA reporting checklist. Available at https://lcm.amegroups.com/article/view/10.21037/lcm-21-50/rc
Peer Review File: Available at https://lcm.amegroups.com/article/view/10.21037/lcm-21-50/prf
Conflicts of Interest: All authors have completed the ICMJE uniform disclosure form (available at https://lcm.amegroups.com/article/view/10.21037/lcm-21-50/coif). The series “Pharmacology of Chinese Herbal Medicine” was commissioned by the editorial office without any funding or sponsorship. The authors have no other conflicts of interest to declare.
Ethical Statement: The authors are accountable for all aspects of the work in ensuring that questions related to the accuracy or integrity of any part of the work are appropriately investigated and resolved.
Open Access Statement: This is an Open Access article distributed in accordance with the Creative Commons Attribution-NonCommercial-NoDerivs 4.0 International License (CC BY-NC-ND 4.0), which permits the non-commercial replication and distribution of the article with the strict proviso that no changes or edits are made and the original work is properly cited (including links to both the formal publication through the relevant DOI and the license). See: https://creativecommons.org/licenses/by-nc-nd/4.0/.
References
- Lashley T, Schott JM, Weston P, et al. Molecular biomarkers of Alzheimer's disease: progress and prospects. Dis Model Mech 2018;11:dmm031781. [Crossref] [PubMed]
- Selkoe DJ. Alzheimer's disease is a synaptic failure. Science 2002;298:789-91. [Crossref] [PubMed]
- Cabezas-Opazo FA, Vergara-Pulgar K, Pérez MJ, et al. Mitochondrial Dysfunction Contributes to the Pathogenesis of Alzheimer's Disease. Oxid Med Cell Longev 2015;2015:509654. [Crossref] [PubMed]
- Sharma P, Srivastava P, Seth A, et al. Comprehensive review of mechanisms of pathogenesis involved in Alzheimer's disease and potential therapeutic strategies. Prog Neurobiol 2019;174:53-89. [Crossref] [PubMed]
- Angeloni C, Barbalace MC, Hrelia S. Icariin and Its Metabolites as Potential Protective Phytochemicals Against Alzheimer's Disease. Front Pharmacol 2019;10:271. [Crossref] [PubMed]
- Wu B, Feng JY, Yu LM, et al. Icariin protects cardiomyocytes against ischaemia/reperfusion injury by attenuating sirtuin 1-dependent mitochondrial oxidative damage. Br J Pharmacol 2018;175:4137-53. [Crossref] [PubMed]
- Li C, Li Q, Mei Q, et al. Pharmacological effects and pharmacokinetic properties of icariin, the major bioactive component in Herba Epimedii. Life Sci 2015;126:57-68. [Crossref] [PubMed]
- Zhang L, Shen C, Chu J, et al. Icariin decreases the expression of APP and BACE-1 and reduces the β-amyloid burden in an APP transgenic mouse model of Alzheimer's disease. Int J Biol Sci 2014;10:181-91. [Crossref] [PubMed]
- Li F, Zhang Y, Lu X, et al. Icariin improves the cognitive function of APP/PS1 mice via suppressing endoplasmic reticulum stress. Life Sci 2019;234:116739. [Crossref] [PubMed]
- Zhang Y, Kong WN, Chai XQ. Compound of icariin, astragalus, and puerarin mitigates iron overload in the cerebral cortex of Alzheimer's disease mice. Neural Regen Res 2018;13:731-6. [Crossref] [PubMed]
- Li C, Meng P, Zhang BZ, et al. Computer-aided identification of protein targets of four polyphenols in Alzheimer's disease (AD) and validation in a mouse AD model. J Biomed Res 2019;33:101-12. [PubMed]
- Jiang X, Chen LL, Lan Z, et al. Icariin Ameliorates Amyloid Pathologies by Maintaining Homeostasis of Autophagic Systems in Aβ1-42-Injected Rats. Neurochem Res 2019;44:2708-22. [Crossref] [PubMed]
- Zhang ZY, Li C, Zug C, et al. Icariin ameliorates neuropathological changes, TGF-β1 accumulation and behavioral deficits in a mouse model of cerebral amyloidosis. PLoS One 2014;9:e104616. [Crossref] [PubMed]
- Wang Y, Zhu T, Wang M, et al. Icariin Attenuates M1 Activation of Microglia and Aβ Plaque Accumulation in the Hippocampus and Prefrontal Cortex by Up-Regulating PPARγ in Restraint/Isolation-Stressed APP/PS1 Mice. Front Neurosci 2019;13:291. [Crossref] [PubMed]
- Sheng C, Xu P, Zhou K, et al. Icariin Attenuates Synaptic and Cognitive Deficits in an Aβ1-42-Induced Rat Model of Alzheimer's Disease. Biomed Res Int 2017;2017:7464872. [Crossref] [PubMed]
- Li F, Dong HX, Gong QH, et al. Icariin decreases both APP and Aβ levels and increases neurogenesis in the brain of Tg2576 mice. Neuroscience 2015;304:29-35. [Crossref] [PubMed]
- Chen FJ, Liu B, Wu Q, et al. Icariin Delays Brain Aging in Senescence-Accelerated Mouse Prone 8 (SAMP8) Model via Inhibiting Autophagy. J Pharmacol Exp Ther 2019;369:121-8. [Crossref] [PubMed]
- Wu J, Qu JQ, Zhou YJ, et al. Icariin improves cognitive deficits by reducing the deposition of β-amyloid peptide and inhibition of neurons apoptosis in SAMP8 mice. Neuroreport 2020;31:663-71. [Crossref] [PubMed]
- Urano T, Tohda C. Icariin improves memory impairment in Alzheimer's disease model mice (5xFAD) and attenuates amyloid β-induced neurite atrophy. Phytother Res 2010;24:1658-63. [Crossref] [PubMed]
- Zhang L, Shen C, Chu J, et al. Icariin reduces α-synuclein over-expression by promoting α-synuclein degradation. Age (Dordr) 2015;37:9811. [Crossref] [PubMed]
- Zong N, Li F, Deng Y, et al. Icariin, a major constituent from Epimedium brevicornum, attenuates ibotenic acid-induced excitotoxicity in rat hippocampus. Behav Brain Res 2016;313:111-9. [Crossref] [PubMed]
- Li WX, Deng YY, Li F, et al. Icariin, a major constituent of flavonoids from Epimedium brevicornum, protects against cognitive deficits induced by chronic brain hypoperfusion via its anti-amyloidogenic effect in rats. Pharmacol Biochem Behav 2015;138:40-8. [Crossref] [PubMed]
- Jin F, Gong QH, Xu YS, et al. Icariin, a phosphodiesterase-5 inhibitor, improves learning and memory in APP/PS1 transgenic mice by stimulation of NO/cGMP signalling. Int J Neuropsychopharmacol 2014;17:871-81. [Crossref] [PubMed]
- Zhu T, Zhang F, Li H, et al. Long-term icariin treatment ameliorates cognitive deficits via CD4+ T cell-mediated immuno-inflammatory responses in APP/PS1 mice. Clin Interv Aging 2019;14:817-26. [Crossref] [PubMed]
- Chen YJ, Zheng HY, Huang XX, et al. Neuroprotective Effects of Icariin on Brain Metabolism, Mitochondrial Functions, and Cognition in Triple-Transgenic Alzheimer's Disease Mice. CNS Neurosci Ther 2016;22:63-73. [Crossref] [PubMed]
- Nie J, Luo Y, Huang XN, et al. Icariin inhibits beta-amyloid peptide segment 25-35 induced expression of beta-secretase in rat hippocampus. Eur J Pharmacol 2010;626:213-8. [Crossref] [PubMed]
- Crossley NA, Sena E, Goehler J, et al. Empirical evidence of bias in the design of experimental stroke studies: a metaepidemiologic approach. Stroke 2008;39:929-34. [Crossref] [PubMed]
- Nhu NT, Cheng YJ, Lee SD. Effects of Treadmill Exercise on Neural Mitochondrial Functions in Parkinson's Disease: A Systematic Review of Animal Studies. Biomedicines 2021;9:1011. [Crossref] [PubMed]
- Platt TL, Reeves VL, Murphy MP. Transgenic models of Alzheimer's disease: better utilization of existing models through viral transgenesis. Biochim Biophys Acta 2013;1832:1437-48. [Crossref] [PubMed]
- Muratore CR, Rice HC, Srikanth P, et al. The familial Alzheimer's disease APPV717I mutation alters APP processing and Tau expression in iPSC-derived neurons. Hum Mol Genet 2014;23:3523-36. [Crossref] [PubMed]
- Sturchler-Pierrat C, Abramowski D, Duke M, et al. Two amyloid precursor protein transgenic mouse models with Alzheimer disease-like pathology. Proc Natl Acad Sci U S A 1997;94:13287-92. [Crossref] [PubMed]
- Krivinko JM, Koppel J, Savonenko A, et al. Animal Models of Psychosis in Alzheimer Disease. Am J Geriatr Psychiatry 2020;28:1-19. [Crossref] [PubMed]
- Ma N, Pan J, Ye X, et al. Whole-Transcriptome Analysis of APP/PS1 Mouse Brain and Identification of circRNA-miRNA-mRNA Networks to Investigate AD Pathogenesis. Mol Ther Nucleic Acids 2019;18:1049-62. [Crossref] [PubMed]
- St-Amour I, Bosoi CR, Paré I, et al. Peripheral adaptive immunity of the triple transgenic mouse model of Alzheimer's disease. J Neuroinflammation 2019;16:3. [Crossref] [PubMed]
- Oakley H, Cole SL, Logan S, et al. Intraneuronal beta-amyloid aggregates, neurodegeneration, and neuron loss in transgenic mice with five familial Alzheimer's disease mutations: potential factors in amyloid plaque formation. J Neurosci 2006;26:10129-40. [Crossref] [PubMed]
- He L, Deng Y, Gao J, et al. Icariside II ameliorates ibotenic acid-induced cognitive impairment and apoptotic response via modulation of MAPK pathway in rats. Phytomedicine 2018;41:74-81. [Crossref] [PubMed]
- Zola SM, Squire LR, Teng E, et al. Impaired recognition memory in monkeys after damage limited to the hippocampal region. J Neurosci 2000;20:451-63. [Crossref] [PubMed]
- Haass C, Selkoe DJ. Soluble protein oligomers in neurodegeneration: lessons from the Alzheimer's amyloid beta-peptide. Nat Rev Mol Cell Biol 2007;8:101-12. [Crossref] [PubMed]
- Perl DP. Neuropathology of Alzheimer's disease. Mt Sinai J Med 2010;77:32-42. [Crossref] [PubMed]
- Mufson EJ, Ikonomovic MD, Counts SE, et al. Molecular and cellular pathophysiology of preclinical Alzheimer's disease. Behav Brain Res 2016;311:54-69. [Crossref] [PubMed]
- Flood JF, Morley JE. Learning and memory in the SAMP8 mouse. Neurosci Biobehav Rev 1998;22:1-20. [Crossref] [PubMed]
- Morley JE, Kumar VB, Bernardo AE, et al. Beta-amyloid precursor polypeptide in SAMP8 mice affects learning and memory. Peptides 2000;21:1761-7. [Crossref] [PubMed]
- Liu B, Liu J, Shi JS. SAMP8 Mice as a Model of Age-Related Cognition Decline with Underlying Mechanisms in Alzheimer's Disease. J Alzheimers Dis 2020;75:385-95. [Crossref] [PubMed]
- Hanyu H, Sato T, Hirao K, et al. The progression of cognitive deterioration and regional cerebral blood flow patterns in Alzheimer's disease: a longitudinal SPECT study. J Neurol Sci 2010;290:96-101. [Crossref] [PubMed]
- Tanaka M, Fukuyama H, Yamauchi H, et al. Regional cerebral blood flow abnormalities in nondemented patients with memory impairment. J Neuroimaging 2002;12:112-8. [Crossref] [PubMed]
- Zhang W, Mi Y, Jiao K, et al. Kellerin alleviates cognitive impairment in mice after ischemic stroke by multiple mechanisms. Phytother Res 2020;34:2258-74. [Crossref] [PubMed]
- Ma X, Xu W, Zhang Z, et al. Salvianolic Acid B Ameliorates Cognitive Deficits Through IGF-1/Akt Pathway in Rats with Vascular Dementia. Cell Physiol Biochem 2017;43:1381-91. [Crossref] [PubMed]
- Wang J, Yang C, Wang H, et al. A New Rat Model of Chronic Cerebral Hypoperfusion Resulting in Early-Stage Vascular Cognitive Impairment. Front Aging Neurosci 2020;12:86. [Crossref] [PubMed]
- Nunez J. Morris Water Maze Experiment. J Vis Exp 2008;897. [PubMed]
- Li D, Huang Y, Cheng B, et al. Streptozotocin Induces Mild Cognitive Impairment at Appropriate Doses in Mice as Determined by Long-Term Potentiation and the Morris Water Maze. J Alzheimers Dis 2016;54:89-98. [Crossref] [PubMed]
- Michelucci A, Heurtaux T, Grandbarbe L, et al. Characterization of the microglial phenotype under specific pro-inflammatory and anti-inflammatory conditions: Effects of oligomeric and fibrillar amyloid-beta. J Neuroimmunol 2009;210:3-12. [Crossref] [PubMed]
- Saijo K, Crotti A, Glass CK. Regulation of microglia activation and deactivation by nuclear receptors. Glia 2013;61:104-11. [Crossref] [PubMed]
- Li X, Guo Q, Ye Z, et al. PPAR γ Prevents Neuropathic Pain by Down-Regulating CX3CR1 and Attenuating M1 Activation of Microglia in the Spinal Cord of Rats Using a Sciatic Chronic Constriction Injury Model. Front Neurosci 2021;15:620525. [Crossref] [PubMed]
Cite this article as: Kan YX, Su CF, Zhu Z, Liu J, Guan XJ, Iyaswamy A, Cheung KH, Zhang Y, Song JX, Li M. Neuroprotective activities of icariin in rodent models of Alzheimer’s disease: a systematic review and meta-analysis. Longhua Chin Med 2022;5:3.