Acupuncture’s neuroanatomic and neurophysiologic basis
Introduction
Bian stones (“stone needles”) dating to the New Stone Age (10000–40000 BCE) are believed to have been used clinically to incise abscesses, perform bloodletting, and regulate Qi circulation (1,2). Inscriptions on bones and tortoise shells dating to the Shang Dynasty era (1556–1046 BCE) illustrate acupuncture and moxibustion practice (1,2). The Huangdi Neijing (Neijing) treatise, dating from the Warring States period in China (475–221 BCE), first compiled the foundations of Traditional Chinese Medicine (including acupuncture) that reflect thousands of years of accumulated clinical knowledge and experience (3).
Though the Neijing does not describe an anatomic basis for the Primary Channels and the Classical acupuncture points that exist on them, it does provide a clue in Article 3.1.3: “… the twelve main channels run latently in the muscles… they are deep and invisible… what can be seen is usually where the foot Greater Yin-Spleen Channel passes the medial malleolus… it is not hidden there” (3). Other historical evidence comes from ten Rhijne’s 1683 dissertation on his observations of acupuncture practice in Nagasaki in which he noted Chinese and Japanese acupuncturists used the terms artery, vein, and nerve interchangeably when discussing the acupuncture channels—this description is consistent with the anatomic fact that arteries, veins and nerves course through the body together as neurovascular bundles in the intermuscular fascial layers (4). At the medial ankle region, the great saphenous vein, which is accompanied by the saphenous nerve, is visible (5,6). The distribution of the Spleen Channel in the distal lower leg and foot mirrors the distribution of the saphenous nerve there (Figure 1), consistent with the Neijing description of the visible portion of the Spleen Channel at the ankle (3,5,6).
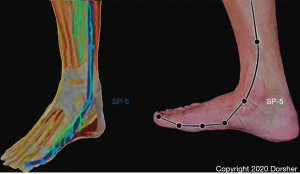
In the last century, technical advances in both the basic biomedical sciences (including anatomy, biochemistry, pharmacology, electrophysiology, and radiology) and clinical research methodologies have led to publication of an extensive body of basic science and clinical acupuncture research on the topics of acupuncture anatomy, physiology, and clinical effects. Indeed, acupuncture research publications have grown exponentially over the past two decades (7). This scientific literature demonstrates that the beneficial clinical effects of acupuncture derive from the activation of peripheral nerves by needling, with resulting secondary modulatory effects on the peripheral/central nervous (including limbic and autonomic structures), immune, and endocrinologic systems.
Theories of acupuncture mechanisms
Over the millennia since the Neijing was published, a number of different theories of what structures/mechanisms underlie acupuncture’s beneficial clinical effects have been proposed. These theories can be broken down into two major categories: anatomic and non-anatomic mechanisms (Table 1).
Table 1
Anatomic theories | Non-anatomic theories |
---|---|
Bonghan circulatory system (8) | Biophotons (9) |
Cutaneous intrinsic visceral afferent nervous system (10) | Electromagnetic field (11,12) |
Hypodermic interstitial fluid migration channels (13) | Neuroimmune-endocrine (14) |
Perimuscular interstitial connective tissues (15) | |
Peripheral nerve (16-19) | |
Perivascular spaces (20) | |
Vascular system (21,22) |
The basic science literature serves to exclude proposed acupuncture mechanisms that involve circulatory and/or humoral systems (8,13,14,20-22). Simply, a tourniquet of an extremity does not affect the efficacy of its acupuncture points for analgesia in humans or animals (23-25). Further, Lu et al. showed that isolation of circulation to Zusanli (ST-36) acupoint from the general circulation does not affect its clinical analgesic effects (24). These studies demonstrate that acupuncture’s afferent signaling mechanisms do not involve the vascular system or humoral factors. Bonghan circulatory system, hypodermic interstitial fluid migration channel, perivascular space, and vascular signaling mechanisms are not plausible anatomic or physiologic mechanisms for acupuncture signaling as none of these mechanisms can be reconciled with the tourniquet studies’ results—all of these anatomic, afferent signaling pathways for acupuncture would be obliterated/blocked by proximal tourniquet application (8,13,14,20-22).
The interstitial fascia model of acupuncture mechanisms also is inconsistent with the above cited tourniquet studies, as the fascia model experimental studies’ results showed that estimated perimuscular connective tissue displacements 4–6 cm from the needle stimulation site were only ~5 micrometers (<1/10 the width of a human hair) and measured torques along the interstitial fascial plane with needling were only 200 μN-m (~2×10−5 kg-m) maximum, which would be of insufficient magnitude to pass the high interstitial tissue compression forces at the proximal limb locations (far distant from the acupuncture needle insertion site) where the tourniquet studies applied those circumferential limb forces (15,23-27). Second, the perimuscular interstitial fascia model of acupuncture cannot explain the results from large, scientifically rigorous acupuncture clinical trials that showed clinically and statistically significant positive results in their control groups that utilized non-penetrating “needling” or minimal superficial needling at the acupoints examined in those studies—these trials’ “sham” needles were not inserted near perimuscular fascia and neither study utilized needle rotation that would “wind” that fascia around their “sham” needles (15,28,29). Finally, the interstitial fascia model also cannot explain the clinical benefits seen with laser acupuncture, as laser energy produces no displacement of the perimuscular fascia (30).
The non-anatomic models of acupuncture mechanisms, specifically biophoton and electromagnetic field acupuncture signaling, also cannot be reconciled with acupuncture basic science and clinical studies (9,11,12). Anesthetic blockade of nerves suppling acupuncture points abolished their pain and non-pain clinical effects (23,31,32). Anesthetic blockade of the brachial plexus eliminates typical brain fMRI activity produced by Hegu (LI-4) stimulation (33). Sectioning the peroneal or sciatic nerve (but not sectioning the femoral or tibial nerve) eliminated or nearly eliminated the analgesic effect of stimulating ST-36 in rats (24). Sectioning the sciatic or femoral nerve in rats abolishes the increased pyloric motility induced by hindpaw stimulation, whether acupuncture stimulation occurs at skin and/or muscle tissue level (34). Anesthetic nerve blockade or nerve sectioning would not eliminate biophoton or electromagnetic field generation, so those two theories are not plausible explanations for acupuncture’s afferent signaling mechanisms.
Thus, the acupuncture basic science and clinical trial literature by itself demonstrates that the only mechanism of acupuncture’s afferent signaling that is consistent with its basic science and clinical trial literature is neurologic (10,16-19). There is overwhelming basic and clinical scientific evidence supporting a neuroanatomic/neurophysiologic model of acupuncture’s mechanisms, which will now be detailed in this review.
Anatomic evidence of acupuncture’s neural based mechanisms
There is microscopic and macroscopic anatomic evidence that peripheral nerves are stimulated at Classical acupoints to produce acupuncture’s clinical effects. Histologic studies of acupuncture points in humans and animals demonstrate the presence of small neurovascular bundles containing A-delta (Aδ) and C nerve fibers penetrating through pores formed by bone, muscle, and/or fascia (35-37). Antidromic sensory nerve stimulation studies in rats demonstrated that the receptive fields of Aδ and C nerve fibers were concentrated either at the sites of acupuncture points or along the course of acupuncture channels, indicating that peripheral anatomic sites demonstrating a high density of nerve endings (Aδ and C fiber nerve terminals) are closely associated anatomically with Classical acupoint locations (38).
Macroscopically, analysis utilizing all major print anatomic references including those of Clemente, Netter, Gray, Grant, and Chen as well as multiple contemporary 3D digital anatomic references demonstrate that Classical acupoint locations are anatomically proximate to cranial or peripheral nerves, or their branches (5,6,39-47). These anatomic references include acupoint—specific anatomic analyses with Chen’s anatomic dissection based acupoint anatomy reference atlas that covers the anatomy of all Classical acupoints, plus the anatomy for acupuncture digital 3D anatomy reference—a digitized human cadaver demonstrates acupuncture needle 3D trajectory for 88 commonly used Classical acupoints (41,43). The Shanghai College of Tradition Medicine acupuncture reference on page 111 documents that in 1960 its Anatomical Teaching and Research Group in a study of 324 acupuncture points, found that 323 were supplied by nerves with “microscopy showing all layers of skin and muscle tissues at acupuncture sites contained numerous and varied nerve branches, plexi, and endings” and this reference documents as well “a particularly close relationship between the paths of the channels on the limbs and the pathways of peripheral nerves” (19). Examples of these relationships demonstrated on a body region basis are as follows.
Figure 2 shows the anatomic relationship of Classical acupoints and Primary Channels in the head region to cranial and spinal nerve branches there. Figure 2A shows that for the frontomaxillary region, Classical acupoints are proximate to trigeminal nerve branches there, with the Bladder Channel distribution mirroring that of the supratrochlear nerve, the Gallbladder Channel distribution mirroring that of the supraorbital nerve, and the Stomach Channel distribution mirroring those of infraorbital nerve branches. Meltz and Chiang confirmed these anatomic relationships by cadaveric and electrical stimulation analysis for 26 classical and 10 “extra” head region acupoints located in the trigeminal nerve distribution, as does Chen’s acupoint cross-sectional anatomy atlas (41,48). Figure 2B shows anatomic evidence of anastomosis of the supratrochlear nerve (a cranial nerve branch) with the greater occipital nerve (a spinal nerve branch) near the vertex—note the combined distributions of these two nerves replicates the distribution of the Bladder Channel from the medial eyebrow over the medial scalp to the upper cervical spine (5).
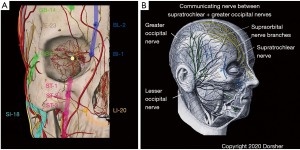
The close anatomic relationship of Classical acupoints and the Primary Channels to the peripheral nervous system (spinal nerve branches) in the trunk region is likewise evident from print and digital anatomic references, including the acupoint cross-sectional anatomy atlas of Chen, as shown in Figure 3 (41).
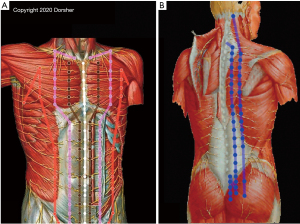
Figure 3B demonstrates the relationship of the Bladder Channel acupoint locations in the posterior trunk to spinal nerve branches, with inner Bladder Channel acupoint locations being anatomically proximate to intermediate branches of the dorsal rami of the spinal nerves, and outer Bladder Channel acupoint locations being anatomically proximate to lateral branches of the dorsal rami. Figure 3A demonstrates the close anatomic relationship of anterior trunk Classical acupuncture point locations to branches of the ventral rami of the spinal nerves for Spleen, Stomach, Kidney, and Conception Vessel Classical acupoints. Conception Vessel acupoints (white) receive bilateral innervation and Kidney acupoints (brown) ipsilateral innervation from branches of the anterior cutaneous branches of the spinal nerves’ ventral rami, where they penetrate the fascia in the medial anterior trunk to provide cutaneous innervation (5,6). Stomach acupoints (pink) receive innervation from more lateral branches of the anterior cutaneous branches of the spinal nerves’ ventral rami, while Spleen acupoints (orange) receive innervation from anterior branches of the lateral cutaneous branches of the spinal nerves’ ventral rami.
The thorax is (normally) anatomically larger than the abdomen, which along with the presence of the sternum in the midline, anatomically leads to more lateral locations of the anterior cutaneous branches of the spinal nerves’ ventral rami in the thorax compared to those in the abdominal region (5,6). Consistent with that anatomic finding, Figure 3A also demonstrates that Kidney and Stomach Classical acupoints’ described locations are more laterally positioned in the thorax than the abdomen (19,49). Note the Primary, Conception, and Governing Vessel Channels’ distributions course perpendicular to the transverse orientation of the spinal nerves in the trunk, which likely relates to the differences in embryologic development of the trunk, extremity, and head/neck regions (50-52).
The bilateral innervation of the midline in the anterior and posterior trunk may provide an anatomic basis for the Conception Vessel Channel being considered the “Yin of the Yin” and the Governing Vessel Channel the “Yang of the Yang” (19,49).
The close anatomic relationship of Classical acupoint locations and Primary Channels to peripheral nerves in the upper and lower extremities is also confirmed by standard and digital anatomy resources, including the acupoint cross-sectional anatomy atlas of Chen and Anatomy for Acupuncture, as shown in Figure 4 (41,43). Recent cadaveric confirmation of Lung and Kidney acupoints’ relationships to peripheral nerves has provided even further anatomic evidence of acupoint-nerve correspondences (16,53).
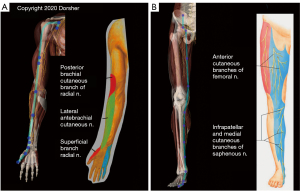
Figure 4A demonstrates the clear anatomic correspondences of Lung Channel acupoint locations and the Lung Channel distribution itself to the distribution of the lateral cord of the brachial plexus and its distal branches (the musculocutaneous and lateral antebrachial cutaneous nerves) in the upper extremity from the anterior pectoral region all the way to the base of the thumb. There is similar compelling overlap of the distribution of the femoral nerve and its distal saphenous nerve sensory branch to the distribution of the Spleen Channel and Spleen acupoint locations from the groin all the way to the base of the great toe, as shown in Figure 4B. Further, the Spleen Channel (dark blue) in the lower extremity has 6 directional changes of 10–40 degrees (mean 20 degrees) along its described course from the groin to the great toe. These directional changes accurately reflect those of the femoral and saphenous nerve as shown in Figure 4B. The statistical odds that the Spleen Channel would coincidentally follow the femoral/saphenous nerve directional changes throughout the lower extremity is ~1 in 34 million, further evidence that these close Classic acupoint to peripheral nerve anatomic relationships are not due to chance.
The close relationship of many Classical acupuncture point locations to peripheral nerves had been documented decades ago in acupuncture references including the Shanghai College of Traditional Medicine text, but the development of 3D anatomic software in the last 15 years markedly enhances to the ability to confirm these anatomic relationships. Primal Picture’s 3D software “Anatomy for Acupuncture” (based on a digitized human cadaver) shows the anatomic trajectory of 88 commonly used Classical acupoints in clinical practice (19,43). This digital acupuncture anatomy model demonstrates the close anatomic proximity of these Classical acupoints to peripheral nerve branches, whose distributions also mirror those of these acupoints’ Primary Channels (43).
da Silva has shown the anatomic proximity of head and neck acupuncture points to the Vagus nerve and its branches, as well as their physiologic/clinical similarities (54). Dimitrova found in randomized controlled trials of acupuncture in treating neuropathies, the acupoints used in those trials were all closely anatomically related to peripheral nerves and those points’ acupuncture indications were consistent with treating neuropathic pain (55).
Physiologic evidence of acupuncture’s neural based mechanisms
Beyond the comprehensive evidence outlined above of the anatomic correspondence of Classical acupoints and peripheral nerves and their branches, the acupuncture literature also provides overwhelming physiologic evidence of the fundamental involvement of the nervous system in transducing acupuncture’s clinical effects.
The physiologic importance of the Aδ and C nerve fibers, which are anatomically present at acupuncture point locations as previously discussed, in transducing acupuncture’s clinical effects is well documented in the literature. Optimal acupuncture stimulates both Aδ and C nerve fibers in producing its analgesic effects. Experimental evidence demonstrates that in vivo, Aδ nerve fiber stimulation is required to produce analgesia outlasting the needle stimulation, while C-fiber stimulation is required to achieve descending pain inhibition (56,57). Analgesia produced by manual acupuncture is similar to that evoked by activation of the diffuse noxious inhibitory control (DNIC) system, which is mediated by Aδ and C-type afferents (58,59). Electrical stimulation of acupuncture needles stimulates C-fibers; and while sufficient electrical stimulation intensity to activate Aβ fibers produces some degree of acupuncture analgesia, more potent analgesia occurs if the stimulation intensity is sufficient to activate Aδ afferent fibers (60,61).
The physiologic importance of peripheral nerves in afferent acupuncture signaling is also well documented in the acupuncture literature. Clinically, acupuncture is ineffective when applied to areas of skin affected by postherpetic neuralgia, where pinprick sensation (subserved by Aδ and C fibers) is usually absent (62,63). Capsaicin application to nerves selectively blocks conduction in Aδ and C fibers—capsaicin applied to the bilateral sciatic nerves before manual acupuncture stimulation of ST-36 completely abolished its analgesia effects in rats (59). In humans, anesthetic block of the nerves innervating the location of LI-4 abolishes its physiologic effect for pain reduction, while median nerve anesthetic block abolishes the anti-emetic effect of Neiguan (PC-6) (31,32). Anesthetic blockade of the brachial plexus abolishes the patterns of brain activation induced by electroacupuncture (EA) at LI-4 (33). Sectioning the peroneal or sciatic nerve (but not sectioning the femoral or tibial nerve) eliminated or nearly eliminated the analgesic effect of stimulating ST-36 in rats (24). Sectioning the sciatic and femoral nerves in rats abolishes the increased gastric motility produced with hindpaw acupuncture-like stimulation (34).
Historical experience has suggested that achieving deqi (“arrival of qi”) sensation when inserting a needle at an acupuncture point is important to achieve optimal therapeutic results from acupuncture treatment (23,64-66). Hui et al. scientifically examined this deqi sensation, and determined that the majority of the deqi sensations, such as aching, soreness, dull pain, and warmth involve the slower conducting Aδ and C fibers (67). Gu et al. demonstrated that the BOLD signal of the thalamus on fMRI correlated significantly with acupuncture (deqi) sensation score during EA at Hegu (LI-4), and that specific brain fMRI changes noted in the bilateral thalamus, basal ganglia, cerebellum and left putamen regions with LI-4 stimulation were eliminated by ipsilateral brachial plexus anesthetic block (33). This experimental data is consistent with afferent Aδ and C fiber neural signaling with acupoint stimulation, with the degree of neural stimulation of those fibers proportional to the needle (deqi) sensation achieved.
The other essential component of the neuroanatomic basis of Classical acupuncture points is their sympathetic autonomic nervous system (SANS) innervation. Postganglionic sympathetic nerve fibers from thoracolumbar sympathetic ganglia (prevertebral or paravertebral) compromise 20% or more of spinal nerve fibers and also invest the adventitial layers of the arteries that accompany spinal nerves and their branches to the extremities (39,68,69). The sympathetic innervation of the arteries, however, only appears to regulate vascular tone of the vessel it invests (69). There is somatotopic organization of nerve fibers within distal peripheral nerve fascicles, which particularly in smaller fascicles contain a bundled organization of sympathetic nerves (70,71). This provides a plausible anatomic basis for acupoints’ differing effects on autonomic (visceral) functions.
The neuroanatomy/neurophysiology of the Back Shu points (Figure 5) also corroborates the neural basis of acupuncture mechanisms.
Each of these Back-Shu Classical acupoint locations is innervated by the corresponding dorsal ramus of the spinal nerve at its segmental level. As shown in Table 2, the Back-Shu acupoints exist at the same spinal segmental levels as the known anatomic segmental SANS outflow to their respective Organs for 9 of the 12 Back-Shu points (19,49,72,73).
Table 2
Organ | Shu point | Spinal level | Sympathetic segmental innervation per Bonica (72) + Beal (73) | Osteopathic level |
---|---|---|---|---|
Lung | BL-13 | T3 | T2-T7 (T2-T5) | T3-T9 |
Pericardium | BL-14 | T4 | T1-T4 (T1-T5)? | C8, T1-T8? |
Heart | BL-15 | T5 | T1-T4 (T1-T5) | C8, T1-T8 |
Liver | BL-18 | T9 | T6-T9 (T5-T10) | T6-T11 |
Gallbladder | BL-19 | T10 | T5-T9 (T7-T10) | T6-T11 |
Spleen | BL-20 | T11 | T6-T10 (T5-T11) | T7-T10 |
Stomach | BL-21 | T12 | T6-T9 (T5-T11) | T7-T10 |
Triple heater (?adrenal) | BL-22 | L1 | Cortex T6-L2, medulla T11-L1 | |
Kidney | BL-23 | L2 | T11-L1 (T10-L2) | T9-L2 |
Large intestine | BL-25 | L4 | Proximal 2/3 T10-L1 (T6-L1), distal 1/3 L1-L2 (T6-L2), (white-afferent S2-S4) | T9-L1 |
Small intestine | BL-27 | S1 | T6-T11 (T6-T12) | T6-T11 duodenum + jejunum |
Bladder | BL-28 | S2 | T11-L2 (white-afferent S2-S4), S2-S3 parasympathetic |
The Back-Shu points of the Stomach and Large Intestine Organs are near (one and two spinal segments caudal, respectively) to their described SANS innervation (72,73). The Back-Shu point of the Small Intestine Organ is located at the S1 level and the Bladder Organ at S2, far caudal to the described SANS outflow to this Organ (Table 2). Note the Bladder Back-Shu point is at the spinal level of its known parasympathetic outflow, however. Given the 14 potential spinal levels involved in SANS innervation (12 thoracic, 2 lumbar) of the trunk organs, the statistical odds that these anatomic/physiologic correspondences would occur by chance is ~1 in 5 billion (1/149). Note these Back-Shu points are the same spinal levels that manual medicine practitioners mobilize clinically to selectively influence internal organ function (73).
Thus, the acupuncture basic science literature provides compelling anatomic and physiologic evidence that Classical acupuncture points are neurologically based.
Acupuncture’s central nervous system (CNS) mechanisms
The acupuncture basic science literature also documents the essential involvement of CNS ascending/descending pathways and central processing of afferent neural signaling from acupuncture points in producing acupuncture’s clinical effects.
Acupuncture analgesia requires intact spinal cord ascending pain pathways in its ventrolateral funiculus. Needling the affected side in hemiplegic or below level of injury in paraplegic patients has no analgesic effect on painful stimulation of unaffected limbs (31). In patients with syringomyelia, when the anterior commissure and dorsal horn of the spinal cord are damaged, pain and temperature sensation deficits are accompanied by reduced or abolished acupuncture effects and ability to feel deqi sensation (74). Damage to the posterior columns experimentally (or clinically as in tabes dorsalis) has little effect on acupuncture analgesia, while unilateral sectioning of the ventrolateral funiculus of the spinal cord (carrying pain and temperature information rostrally) nearly eliminates acupuncture analgesia in the contralateral (but not ipsilateral) limb (75).
Spinal cord and brain activation of endogenous descending pain inhibition mechanisms also contribute to acupuncture analgesia. Acupuncture produces analgesia in non-segmental fashion likely through C-fiber mediated activation of descending pain inhibition including activation of the diffuse noxious inhibitory control (DNIC) system, which is mediated by Aδ and C-type afferents (56,58,59,76). Toda et al. documented that stimulation of forelimb paw point LI-4 in rats reduces experimentally induced dental pain, and Kim et al. have demonstrated that stimulation of a forelimb acupoint SI-6 in rats reduces lower limb dorsal horn neuronal activity (pain) caused by experimental pain from ankle sprain (60,77). Acupuncture also produces both ipsilateral and contralateral analgesia experimentally in humans (78). Intact central nervous pathways (spinal cord and brain) are necessary for these analgesic effects to occur (77,78).
These spinal cord and brain pathways are involved in the mechanisms of acupuncture’s non-pain effects as well. In rats, sectioning the bilateral femoral and sciatic nerves, or sectioning the bilateral Vagus nerves, abolishes the increased pyloric motility induced by hindpaw stimulation, whether acupuncture stimulation occurs at skin and/or muscle tissue level (34). Noguchi and Hayashi demonstrated in rats that increased gastric acid secretion produced by EA of the hindlimb was abolished by sciatic nerve denervation or by Vagus nerve sectioning (79). These studies illustrate that afferent acupuncture point stimulation in the hindlimb is processed in the CNS, with clinical effect on gastric motility and acid secretion occurring through the descending Vagus nerve pathway.
Cheng and Pomerantz demonstrated that acupuncture stimulation causes release of endorphins and enkephalins that are fundamentally important in acupuncture’s analgesic response, as naloxone blocks or significantly attenuates acupuncture analgesia (80). The release of these endogenous opioids results from corticotropin-releasing hormone (CRH) release from the hypothalamus that in turn releases pro-opiomelanocortin (from which endorphins and enkephalins derive) from the anterior and central pituitary gland (80). Lesions of arcuate nucleus of the hypothalamus abolish acupuncture analgesia, and experimental reduction of pituitary endorphin suppresses acupuncture analgesia (81,82). Endorphin levels rise in blood and cerebrospinal fluid (CSF) during acupuncture analgesia - this acupuncture analgesia can be transmitted to a second animal by CSF transfer and blocked by intra-ventricular opiate antagonist administration (31,83-86). In human experiments, acupuncture analgesia produces bilateral analgesia consistent with these central mechanisms including pituitary release of endorphins and enkephalins (78).
Acupuncture also modulates hypothalamic pituitary adrenal (HPA) axis activity in inflammatory pain states. EA at Huantiao (GB-30) in an experimental rat paw inflammation study produced a [5–10]-fold increased plasma cortisol levels compared to sham EA controls and also reduced paw inflammation (87). Adrenalectomy eliminated EA inflammation improvement but not its anti-hyperalgesia effect (87). The observed increase in plasma cortisol levels is produced via EA activation of CRH neurons of the hypothalamus’ paraventricular nuclei, which in turn increases ACTH secretion from the anterior pituitary gland two-fold to stimulate the adrenal gland (88).
Functional magnetic resonance imaging studies confirm that stimulation of peripheral Classical acupoints selectively modulates brain structures to produce acupuncture’s analgesic effects consistent with the above physiologic experiment findings. Wu et al. found that LI-4 and ST-36 EA stimulation (but not sham electroacupuncture) produces activation of somatosensory cortex areas subserving pain sensation and localization as well as the hypothalamus (where the process of release of endogenous opioids is initiated) while simultaneously inhibiting limbic system structures including anterior cingulate gyrus and amygdala that subserve the behavioral reaction to pain (89). Manual acupuncture at these points also modulated brain fMRI activity in areas including thalamus, anterior cingulate, insula, and cerebellum, which are also part of the reported neuromatrix of pain (89). Hui et al. found similar results with stimulation of LI-4; and additionally noted that if pain was produced rather than deqi when stimulating LI-4, then the limbic system structures were not inhibited (no reduction of behavioral response to the painful stimulus) (90). This means that proper acupuncture reduces the behavioral reaction to a painful stimulus without interfering with ability to perceive the painful stimulus. Hui et al. further demonstrated acupuncture stimulation produces deactivation of the medial prefrontal, medial parietal and medial temporal lobes and activation of regions in the sensorimotor and paralimbic structures, in a pattern consistent with acupuncture modulating the default mode network and the anti-correlated task-positive network to produce its analgesic effects (91).
Neural basis of acupuncture’s observed clinical (efferent) effects
Acupuncture’s analgesic effects
As previously discussed, the work of Cheng and Pomerantz demonstrated acupuncture stimulation causes release of endorphins and enkephalins from the pituitary, which are experimentally proven to be fundamentally involved in acupuncture analgesia, including the bilateral analgesia produced by unilateral acupuncture (78,80-85). Acupuncture also activates descending spinal cord and brain pain pathways (including the DNIC system) and brain anti-nociceptive networks as described in the previous section (56,75,77,89-91).
Acupuncture’s anti-inflammatory effects
Neurogenic inflammation is defined as inflammation arising from the local release of inflammatory mediators (including substance P, calcitonin gene-related peptide and nitrous oxide) in peripheral nerve terminals of afferent neurons caused by antidromic nerve activity after those afferent neurons are stimulated (92,93). Release of these neuropeptides alters local vascular permeability and helps to initiate pro-inflammatory and immune reactions. Animal models demonstrate acupuncture reduces peripheral inflammation and neurogenic inflammation, producing central and peripheral inhibition of capsaicin-induced inflammation by reducing substance P release in peripheral nerve terminals of nociceptive neurons (94). This appears to be mediated by both serotonergic and endogenous opioid systems (95).
Acupuncture modulation of Vagus nerve efferent activity can regulate local and systemic inflammatory responses. Clinical and experimental evidence demonstrate a negative feedback loop between the autonomic nervous system and the innate immune system called the cholinergic anti-inflammatory pathway (CAP) (96). CAP inhibits the immune system inflammatory response by suppressing cytokine synthesis through the release of acetylcholine in the reticuloendothelial system organs, which include the lungs, spleen, liver, kidneys and gastrointestinal tract (96). Acetylcholine release causes down regulation of pro-inflammatory cytokines by macrophages and other cytokine producing cells to prevent tissue damage (97). Electrical stimulation of the Vagus nerve inhibits macrophage activation and inhibits production of pro-inflammatory cytokines including tumor necrosis factor, IL-1β, IL-6, and IL-18 (98). Vagal nerve stimulation also reduces systemic inflammatory response to endotoxins in sepsis via this peripheral mechanism as well as afferent stimulation of the HPA axis (98). Thus, stimulation of appropriate auricular and/or Channel acupoints can produce local and systemic parasympathetic autonomic nervous system (PANS) modulation that reduces local and systemic inflammation (thus also reducing inflammatory pain).
Acupuncture also modulates HPA axis activity in inflammatory pain states through its effects on ACTH/cortisol secretion, as discussed in the prior section to produce a systemic anti-inflammatory effect (87,88).
Acupuncture’s visceral effects
The relationship of the Back-Shu points to the known segmental SANS outflow of the thoracolumbar spine and the bundled, segmental organization of SANS fibers in distal peripheral nerve fascicles, as previously outlined, are both mechanisms for the selective visceral effects of Classical acupoints seen in clinical practice (68,70,71). Acupuncture selectively modulates efferent Vagus nerve (PANS) visceral activity as well. Animal experiments showed the effects of acupuncture hindlimb stimulation to increase gastric acid secretion and motility is eliminated by vagotomy (34,79). In cats, EA at Pericardium Channel points PC-5 to PC-6 (near volar wrist) has been demonstrated to activate cholinergic Vagal preganglionic neurons in the nucleus ambiguus, at least in part through an enkephalinergic mechanism (99). Human heart rate variability studies demonstrate that stimulation of Hegu (LI-4) produces a statistically significant increase in SANS and PANS activity along with a significant decrease of heart rate (70). Laser stimulation of Taichong (LR-3) in humans significantly lowers blood pressure, heart rate, and heart rate variability compared to sham laser (100).
Neuroanatomic model of acupuncture: implications for needle sensation and the positive randomized clinical trial results seen with “sham” needles
Historical experience has suggested that achieving deqi sensation (subserved by A and C fiber signaling) during acupuncture point needling is important to achieve optimal therapeutic results from acupuncture treatment, though contemporary acupuncture clinical trials have not been able to definitively demonstrate this (23,65-67). Of interest, Gu et al. demonstrated that the intensity of BOLD signal of the thalamus on fMRI correlated significantly with the acupuncture (deqi) sensation score during EA at Hegu (LI-4), suggesting an acupuncture “dose” dependent response of afferent neural signaling to the intensity of needle sensation achieved (33).
Figure 6 shows a neuroanatomic model of acupuncture signaling, that is consistent with the above findings as well as human and canine acupoint histologic studies which demonstrate small neurovascular bundles containing Aδ and C nerve fibers penetrating through pores formed by bone, muscle and/or fascia (35-37). This model is also consistent with Kim et al.’s findings that cutaneous neurogenic inflammatory spots on the skin associated with visceral disorders corresponded to traditional acupuncture points (101).
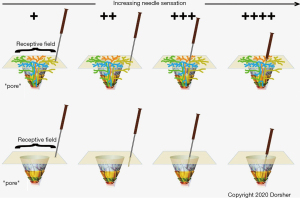
The top portion of Figure 6 illustrates four such nerve fibers (gold, orange, green and blue) at one acupoint “pore”. Though the fibers give some innervation to the subcutaneous tissues as shown, these Aδ and C fibers mostly innervate their cutaneous receptive fields as shown. The higher density of nerve branches cutaneously fits with skin being more sensitive to needling than subcutaneous tissues. As shown in Figure 6A, cutaneous pressure from acupressure or a non-penetrating “sham” needle will produce some degree of afferent neural signaling from stimulation of the “gold” nerve terminals. With needling into the subcutaneous tissue locally as shown in Figure 6B , deeper sensory nerves will also be stimulated producing a larger afferent neural signal. If, as in Figure 6C, the acupuncture needle is inserted closer to the acupuncture “pore”, other nerve fascicles (orange and blue) will be stimulated leading to a much larger afferent neural signal. Finally, Figure 6D shows that if the acupuncture needle tip reaches the “true” acupoint at the actual pore opening, then all 4 nerve fascicles are activated producing the maximal afferent neural signaling. This model is consistent with the results of Gu et al. showing an increase in thalamus activation with increasing needle sensation score, as well as the animal study of Kim et al. showing greater expression of GAP-43 and upregulation of phospho-Erk1/2 signals in dorsal root ganglia sensory neurons with Zusanli (ST-36) stimulation compared to control point stimulation 5–10 mm away (33,102).
This neuroanatomic model provides a plausible explanation for the unexpected clinically and statistically significant positive results of “sham” acupuncture in large randomized clinical trials (though subjects receiving verum acupuncture had somewhat better outcomes). Cherkin et al.’s acupuncture for lumbar pain trial used non-penetrating “needling” (toothpick in a guide tube) at acupoints as the sham intervention, which would in the neuroanatomic model (Figure 6A) produce Aδ and C fiber afferent stimulation at the acupoint with a resulting physiologic effect for pain reduction (28). Haake et al.’s GERAC acupuncture for lumbar pain randomized controlled trial used “minimal” superficial needling at non-acupoints, most of which were along lumbar and sacral dermatomes/myotomes (29). Per Figure 6B, this minimally penetrating needling would be anticipated to produce greater afferent nerve stimulation than the non-penetrating needle of Cherkin et al.’s study and thus greater physiologic effect for pain reduction (28,29).
Acupuncture point stimulation using laser has demonstrated clinical efficacy in treating pain and other non-pain conditions- this cannot be explained by fascial acupuncture mechanisms, as the light energy does not displace tissue (30). Infrared low level laser energy has been shown to be able to depolarize nerve axons, again consistent with a neural basis of laser acupuncture’s mechanism (103,104).
Discussion
For any proposed theory of acupuncture’s anatomy and/or physiology, the putative mechanism(s) must be compatible with all prior basic and clinical acupuncture research results. The only acupuncture mechanism that is able to do so is a neuroanatomic/neurophysiologic mechanism. Print and digital anatomic references confirm Classical acupoint and Primary Channel anatomic relationships to neural structures. Histologic, neurophysiologic and fMRI studies provide compelling evidence of the fundamental involvement of the peripheral nervous system in transducing and the CNS in processing acupuncture needle stimulation into its efferent (clinical) effects. Acupuncture’s clinical effects ultimately occur through modulation of the innate immunologic, endogenous opioid, hypothalamic-pituitary axis, autonomic, and CAP systems. This in turn results from acupuncture needle stimulation of peripheral nerves, which modulate neurogenic inflammation (via peripheral and central mechanisms) and multiple neural systems including the DNIC network, the Vagus nerve, the autonomic nervous system, and the CNS including its default mode and anti-correlated task-positive networks. Acupuncture influence on viscera can be understood through the segmental innervation of the Back-Shu points, the somatotopic/bundled organization of sympathetic nerve fibers in distal peripheral nerve fascicles, and acupuncture’s modulation of autonomic nervous system (including Vagus nerve) activity/balance.
The neuroanatomic/neurophysiologic model of acupuncture mechanism serves to reinforce the clinical brilliance of the founders of acupuncture and traditional Chinese medicine 2200+ years ago—these acupuncture scientist/clinicians described essential aspects of human neurophysiology despite not having 20th century technology available to them to be able to study autonomic, peripheral, and CNS functions. This neuroanatomic and neurophysiologic model of acupuncture suggests that Classical acupoints and the Channels they exist on offers a mapping of the human autonomic nervous system in the head, trunk, and extremities that is very non-linear compared to the dermatome and myotome distributions. This autonomic mapping of the human nervous system has largely yet to be “discovered” (rediscovered) by allopathic medicine some 2 millennia later, though there have been contemporary clinical introductions of “seasickness” wrist bands stimulating the Neiguan (PC-6) region for treatment of nausea/vomiting, as well as the PTNS (posterior tibial nerve stimulator) device currently used to treat urge incontinence (105,106). This PTNS device electrically stimulates the medial leg near San Yin Jiao (SP-6), whose acupuncture clinical indications include difficult urination, incontinence, and enuresis (19,49). The Cefaly device for headache stimulates scalp over Yintang, Cuanzhu (BL-2) and Yangbai (GB-14), which are all used to treat headaches in the acupuncture tradition (19,49,107). All these devices are clearly “rediscovering” the clinical benefits of acupuncture described 2000+ years ago.
“Nothing is new”.
Conclusions
The acupuncture literature provides overwhelming anatomic and physiologic evidence that all of acupuncture’s beneficial analgesic and non-analgesic effects can be explained through neuroanatomic and neurophysiologic mechanisms—that is, acupuncture stimulation of specific peripheral nerve locations (acupoints) produces its clinical effects. A neural based model of acupuncture is the only model that is consistent with acupuncture basic science and clinical literature.
Acknowledgments
Funding: None.
Footnote
Peer Review File: Available at https://lcm.amegroups.com/article/view/10.21037/lcm-21-48/prf
Conflicts of Interest: Both authors have completed the ICMJE uniform disclosure form (available at https://lcm.amegroups.com/article/view/10.21037/lcm-21-48/coif). The authors have no conflicts of interest to declare.
Ethical Statement: The authors are accountable for all aspects of the work in ensuring that questions related to the accuracy or integrity of any part of the work are appropriately investigated and resolved.
Open Access Statement: This is an Open Access article distributed in accordance with the Creative Commons Attribution-NonCommercial-NoDerivs 4.0 International License (CC BY-NC-ND 4.0), which permits the non-commercial replication and distribution of the article with the strict proviso that no changes or edits are made and the original work is properly cited (including links to both the formal publication through the relevant DOI and the license). See: https://creativecommons.org/licenses/by-nc-nd/4.0/.
References
- Xinnong C. editor. Chinese Acupuncture and Moxibustion 3rd Edition, 19th Printing. Beijing Foreign Language Press; Dec. 2018.
- China.Org.CN. A Brief History of Chinese Acupuncture and Moxibustion. Available online: http://www.china.org.cn/archive/2006-10/27/content_1185663.htm. Accessed 7/30/2021.
- Zhu M (translator). The medical classic of the yellow emperor. Beijing: Foreign Languages Press, 2001.
- Baldry PE. Acupuncture, trigger points, and musculoskeletal pain. 3rd edition. London: Elsevier, 2005:14-16.
- Clemente CD. Anatomy: a regional atlas of the human body. 2nd ed. Baltimore: Urban & Schwarzenberg, 1981. ISBN-13: 9780806703220.
- Netter FH. Atlas of human anatomy. Summit: Ciba-Geigy, 1989.
- Ma Y, Dong M, Zhou K, et al. Publication trends in acupuncture research: a 20-year bibliometric analysis based on. PLoS One Dec 4, 2016; Available online:
10.1371/journal.pone.0168123 10.1371/journal.pone.0168123 - Soh KS. Bonghan circulatory system as an extension of acupuncture meridians. J Acupunct Meridian Stud 2009;2:93-106. [Crossref] [PubMed]
- Gomes LR, Leão P. Recent Approaches on Signal Transduction and Transmission in Acupuncture: A Biophysical Overview for Medical Sciences. J Acupunct Meridian Stud 2020;13:1-11. [Crossref] [PubMed]
- Silberstein M. The cutaneous intrinsic visceral afferent nervous system: a new model for acupuncture analgesia. J Theor Biol 2009;261:637-42. [Crossref] [PubMed]
- Dale RA. Demythologizing acupuncture Part 1. The scientific mechanisms and the clinical uses. Alternative & Complementary Therapies Journal 1997;1:125-31. [Crossref]
- Volf N, Ferdman L. The mechanisms of immunomodulation in acupuncture. Int J Complement Alt Med 20179:00320.
- Li HY, Yang JF, Chen M, et al. Visualized regional hypodermic migration channels of interstitial fluid in human beings: Are these ancient meridians? J Altern Complement Med 2008;14:621-8. [Crossref] [PubMed]
- Zhang K, Guo XM, Yan YW, et al. Applying Statistical and Complex Network Methods to Explore the Key Signaling Molecules of Acupuncture Regulating Neuroendocrine-Immune Network. Evid Based Complement Alternat Med 2018;2018:9260630. [Crossref] [PubMed]
- Langevin HM, Yandow JA. Relationship of acupuncture points and meridians to connective tissue planes. Anat Rec 2002;269:257-65. [Crossref] [PubMed]
- Chiang P. What is the point of acupuncture? Med Acup 2015;27:67-9. [Crossref]
- Dorsher P. The Neuroanatomic Basis of the Acupuncture Principal Meridians. Nat Prec (2009). Available online: https://doi.org/
10.1038/npre.2009.3795.1 - Longhurst JC. Defining meridians: a modern basis of understanding. J Acupunct Meridian Stud 2010;3:67-74. [Crossref] [PubMed]
- O’Connor J, Bensky D, editors. Acupuncture: A Comprehensive Text. Chicago (Illinois): Eastland Press; 1981.
- Ma W, Tong H, Xu W, et al. Perivascular space: possible anatomical substrate for the meridian. J Altern Complement Med 2003;9:851-9. [Crossref] [PubMed]
- Hsiao SH, Tsai LJ. A Neurovascular Transmission Model for Acupuncture-induced Nitric Oxide. J Acupunct Meridian Stud 2008;1:42-50. [Crossref] [PubMed]
- Shaw V. Chong meridian an ancient Chinese description of the vascular system? Acupunct Med 2014;32:279-85. [Crossref] [PubMed]
- Chiang CY, Chang CT, Chu HC, et al. Peripheral afferent pathway for acupuncture analgesia. Sci Sin 1973;16:210-7.
- Lu GW. Characteristics of afferent fiber innervation on acupuncture points Zusanli. Am J Physiol 1983;245:R606-R612. [Crossref] [PubMed]
- Musial F, Choi KE, Gabriel T, et al. The effect of electroacupuncture and tramadol on experimental tourniquet pain. Acupunct Med 2012;30:21-6. [Crossref] [PubMed]
- Fox JR, Gray W, Koptiuch C, Badger GJ, Langevin HM. Anisotropic tissue motion induced by acupuncture needling along intermuscular connective tissue planes. J Altern Complement Med 2014;20:290-4. [Crossref] [PubMed]
- Davis RT, Churchill DL, Badger GJ, et al. A new method for quantifying the needling component of acupuncture treatments. Acupunct Med 2012;30:113-9. [Crossref] [PubMed]
- Cherkin DC, Sherman KJ, Avins AL, et al. A randomized trial comparing acupuncture, simulated acupuncture, and usual care for chronic low back pain. Arch Intern Med 2009;169:858-66. [Crossref] [PubMed]
- Haake M, Muller HH, Schade-Brittinger C, et al. German acupuncture trials (GERAC) for chronic low back pain: a randomized, multicenter, blinded, parallel-group trial with 3 groups. Arch Intern Med 2007;167:1892-8. [Crossref] [PubMed]
- Law D, McDonough S, Bleakley C, et al. Laser acupuncture for treating musculoskeletal pain: a systematic review with meta-analysis. J Acupunct Meridian Stud 2015;8:2-16. [Crossref] [PubMed]
- Research Group of Acupuncture Anesthesia. PMC: Effect of acupuncture on the pain threshold of human skin. Chin Med J 1973;3:151-7.
- Dundee JW, Ghaly G. Local anesthesia blocks the antiemetic action of P6 acupuncture. Clin Pharmacol Ther 1991;50:78-80. [Crossref] [PubMed]
- Gu W, Jiang W, He J, et al. Blockade of the brachial plexus abolishes activation of specific brain regions by electroacupuncture at LI4: a functional MRI study. Acupunct Med 2015;33:457-64. [Crossref] [PubMed]
- Sato A, Sato Y, Suzuki A, et al. Neural mechanisms of the reflex inhibition and excitation of gastric motility elicited by acupuncture-like stimulation in anesthetized rats. Neurosci Res 1993;18:53-62. [Crossref] [PubMed]
- Senelar R. Les characteristiques morphologiques des points Chinois. In: Niboyet JEH (ed). Nouveau Traite D'Acupuncture. Paris: Maisonneuve, 1979.
- Kim MC, Nam TC, Kim MK, et al. Histological observation of canine acupoints. The Medical Acupuncture Web Page. Available online: http://www.medvetacupuncture.org/english/articles/ histology/aphistol.html. Accessed 8/8/2021.
- Lou XF, Jiang SH. Anatomical characteristics and classification of acupoints. Zhongguo Zhen Jiu 2012;32:319-23. [PubMed]
- Li AH, Zhang JM, Xie YK. Human acupuncture points mapped in rats are associated with excitable muscle/skin-nerve complexes with enriched nerve endings. Brain Res 2004;1012:154-9. [Crossref] [PubMed]
- Gray H. Anatomy of the Human Body. Available online: https://www.bartleby.com/107/. accessed 8/8/2021.
- Anderson JE. Grant’s Atlas of Anatomy. 7th edition. Baltimore: Williams & Wilkins, 1978:608.
- Chen E. Cross-sectional anatomy of acupoints. Edinburgh: Churchill Livingstone, 1995:244.
- Hillman S. Functional Anatomy. Available through Primal Pictures Anatomy TV. Available online: https://www.anatomy.tv; accessed 6/16/2021.
- Dorsher PT, Cummings M. Anatomy for Acupuncture [DVD]. Primal Pictures: London, UK, 2006. ISBN 9781904369707 available through Primal Anatomy.TV. Available online: https://www.anatomy.tv/anatomytv
- d4Medical. Essential Anatomy 5. Available online: https://3d4medical.com/apps/essential-anatomy-5; accessed 6/16/2021.
- Biodigital. 3D Human Anatomy and Disease. Available online: https://www.biodigital.com; accessed 6/16/2021.
- IMAIOS. e-Anatomy. Available online: https://www.imaios.com/en/e-Anatomy; accessed 6/16/2021.
- Visible Body. Human Anatomy Atlas 2021. Available online: https://www.visiblebody.com; accessed 6/16/2021.
- Meltz L, Ortiz D, Chiang P. The Anatomical Relationship Between Acupoints of the Face and the Trigeminal Nerve. Med Acupunct 2020;32:181-93. [Crossref] [PubMed]
- Deadman P, Al-Khafaji M, Baker K. A manual of acupuncture. East Sussex Journal of Chinese Medical Publications 1998;ISBN-13:9780951054659.
- Dorsher PT. Neuroembryology of the Acupuncture Principal Meridians: Part 1. The Extremities. Med Acupunct 2017;29:10-9. [Crossref]
- Dorsher PT. Neuroembryology of the Acupuncture Principal Meridians: Part 2. The Trunk. Med Acupunct 2017;29:77-86. [Crossref]
- Dorsher PT, Chiang P. Neuroembryology of the Acupuncture Principal Meridians: Part 3. The Head and Neck. Med Acupunct 2018;30:80-8. [Crossref] [PubMed]
- Lee M, Longenecker R, Lo S, et al. Distinct neuroanatomical structures of acupoints Kidney 1 to Kidney 8: a cadaveric study. Med Acupunct 2019;31:19-28. [Crossref] [PubMed]
- da Silva MA, Dorsher PT. Neuroanatomic and clinical correspondences: acupuncture and vagus nerve stimulation. J Altern Complement Med 2014;20:233-40. [Crossref] [PubMed]
- Dimitrova A, Murchison C, Oken B. the case for local needling in successful randomized controlled trials of peripheral neuropathy: a follow-up systematic review. Med Acupunct 2018;30:179-91. [Crossref] [PubMed]
- Chung JM, Lee KH, Hori Y, et al. Factors influencing peripheral nerve stimulation produced inhibition of primate spinothalamic tract cells. Pain 1984;19:277-93. [Crossref] [PubMed]
- Chung JM, Fang ZR, Hori Y, et al. Prolonged inhibition of primate spinothalamic tract cells by peripheral nerve stimulation. Pain 1984;19:259-75. [Crossref] [PubMed]
- Bing Z, Villanueva L, Le Bars D. Acupuncture and diffuse noxious inhibitory controls: naloxone-reversible depression of activities of trigeminal convergent neurons. Neuroscience 1990;37:809-18. [Crossref] [PubMed]
- Okada K, Oshima M, Kawakita K. Examination of the afferent fiber responsible for the suppression of the jaw-opening reflex in heat, cold, and the manual acupuncture stimulation in rats. Brain Res 1996;740:201-7. [Crossref] [PubMed]
- Toda K, Ichioka M. Electroacupuncture: relations between forelimb afferent impulses and suppression of jaw-opening reflex in the rat. Exp Neurol 1978;61:465-70. [Crossref] [PubMed]
- Kawakita K, Funakoshi M. Suppression of the jaw-opening reflex by conditioning A-delta fiber stimulation and electroacupuncture in the rat. Exp Neurol 1982;78:461-5. [Crossref] [PubMed]
- Levine JD, Gormley J, Fields HL. Observations on the analgesic effects of needle puncture (acupuncture). Pain 1976;2:149-59. [Crossref] [PubMed]
- Nurmikko T, Bowsher D. Somatosensory findings in postherpetic neuralgia. J Neurol Neurosurg Psychiatry 1990;53:135-41. [Crossref] [PubMed]
- Zhang S, Mu W, Xiao L, et al. Is deqi an indicator of clinical efficacy of acupuncture? A systematic review. Evid Based Complement Alternat Med 2013;2013:750140. [Crossref] [PubMed]
- Kong J, Gollub R, Huang T, et al. Acupuncture de qi, from qualitative history to quantitative measurement. J Altern Complement Med 2007;13:1059-70. [Crossref] [PubMed]
- Zhou W, Benharash P. Significance of “deqi” response in acupuncture treatment: myth or reality. J Acupunct Meridian Stud 2014;7:186-9. [Crossref] [PubMed]
- Hui KKS, Nixon EE, Vangel MG, et al. Characterization of the “deqi” response in acupuncture. BMC Complement Altern Med 2007;7:33. [Crossref] [PubMed]
- Ko HY, Shin YB, Sohn HJ, et al. Unmyelinated fibers in human spinal ventral roots: C4 to S2. Spinal Cord 2009;47:286-9. [Crossref] [PubMed]
- Birch DJ, Turmaine M, Boulos PB, et al. Sympathetic innervation of human mesenteric artery and vein. J Vasc Res 2008;45:323-32. [Crossref] [PubMed]
- Haker E, Egekvist H, Bjerring P. Effect of sensory stimulation (acupuncture) on sympathetic and parasympathetic activities in healthy subjects. J Auton Nerv Syst 2000;79:52-9. [Crossref] [PubMed]
- Tompkins RP, Melling CW, Wilson TD, et al. Arrangement of sympathetic fibers within the human common peroneal nerve: implications for microneurography. J Appl Physiol (1985) 2013;115:1553-61. [PubMed]
- Bonica JJ. Autonomic innervation of the viscera as related to nerve block. Anesthesiology 1968;29:793-813. [Crossref] [PubMed]
- Beal MC. Viscerosomatic reflexes: a review. J Am Osteopath Assoc 1985;85:786-801. [Crossref] [PubMed]
- Cao X. Scientific bases of acupuncture analgesia. Acupunct Electrother Res 2002;27:1-14. [Crossref] [PubMed]
- Chen-yü C, Jen-yi L, Teh-hsing C, et al. Studies on spinal ascending pathway for effect of acupuncture analgesia in rabbits. Sci Sin 1975;18:651-8. [PubMed]
- Liu XA, Jiang MC, Huang PB. The role of afferent C fibers in electroacupuncture of Zusanli point activating nucleus raphe magnus. Sheng Li Xue Bao 1990;42:523-33. [PubMed]
- Kim JH, Kim HY, Chung K, et al. Electroacupuncture reduces the evoked responses of the spinal dorsal horn neurons in ankle-sprained rats. J Neurophysiol 2011;105:2050-7. [Crossref] [PubMed]
- Zaretsky HH, Lee MH, Rubin M. Psychological factors and clinical observations in acupuncture analgesia and pain abatement. J Psychol 1976;93:113-20. [Crossref] [PubMed]
- Noguchi E, Hayashi H. Increases in gastric acidity in response to electroacupuncture stimulation of the hindlimb of anesthetized rats. Jpn J Physiol 1996;46:53-8. [Crossref] [PubMed]
- Cheng RS, Pomeranz BH. Electroacupuncture analgesia is mediated by stereospecific opiate receptors and is reversed by antagonists of type I receptors. Life Sci 1980;26:631-8. [Crossref] [PubMed]
- Wang Q, Mao L, Han J. The arcuate nucleus of hypothalamus mediates low but not high frequency electroacupuncture analgesia in rats. Brain Res 1990;513:60-6. [Crossref] [PubMed]
- Cheng R, Pomeranz B, Yü G. Dexamethasone partially reduces and 2% saline treatment abolishes electroacupuncture analgesia: These findings implicate pituitary endorphins. Life Sci 1979;24:1481-86. [Crossref] [PubMed]
- Peng CH, Yang MM, Kok SH, et al. Endorphin release: a possible mechanism of acupuncture analgesia. Comp Med East West 1978;6:57-60. [PubMed]
- Sjölund B, Ericksson M. Increased cerebrospinal fluid levels of endorphins after electroacupuncture. Acta Physiol Scand 1977;100:382-4. [Crossref] [PubMed]
- Abenyakar S, Boneval F. Increased plasma β-endorphin concentrations after acupuncture: comparison of electroacupuncture, Traditional Chinese acupuncture, TENS and placebo TENS. Acupunct Med 1994;12:21-3. [Crossref]
- Han JS, Terenius L. Neurochemical basis of acupuncture analgesia. Annu Rev Pharmacol Toxicol 1982;22:193-220. [Crossref] [PubMed]
- Li A, Zhang RX, Wang Y, et al. Corticosterone mediates electroacupuncture-produced anti-edema in a rat model of inflammation. BMC Complement Altern Med 2007;7:27. [Crossref] [PubMed]
- Li A, Lao L, Wang Y, et al. Electroacupuncture activates corticotrophin-releasing hormone-containing neurons in the paraventricular nucleus of the hypothalamus to alleviate edema in a rat model of inflammation. BMC Compl Altern Med 2008;8:20. [Crossref]
- Wu MT, Sheen JM, Chuang KH, et al. Neuronal specificity of acupuncture response: a fMRI study with electroacupuncture. Neuroimage 2002;16:1028-37. [Crossref] [PubMed]
- Hui KK, Liu J, Makris N, et al. Acupuncture modulates the limbic system and subcortical gray structures of the human brain: evidence from fMRI studies in normal subjects. Hum Brain Mapp 2000;9:13-25. [Crossref] [PubMed]
- Hui KK, Marina O, Claunch JD, et al. Acupuncture mobilizes the brain's default mode and its anti-correlated network in healthy subjects. Brain Res 2009;1287:84-103. [Crossref] [PubMed]
- Kajekar R, Moore PK, Brain SD. Essential role for nitric oxide in neurogenic inflammation in rat cutaneous microcirculation: Evidence for an endothelium-independent mechanism. Circulation Research 1995;76:441-7. [Crossref] [PubMed]
- Richardson JD, Vasko MR. Cellular mechanisms of neurogenic inflammation. J Pharmacol Exp Ther 2002;302:839-45. [Crossref] [PubMed]
- Ceccherelli F, Gagliardi G, Ruzzante L, et al. Acupuncture modulation of capsaicin-induced inflammation: effect of intraperitoneal and local administration of naloxone in rats: A blinded controlled study. J Altern Complement Med 2002;8:341-9. [Crossref] [PubMed]
- Ceccherelli F, Gagliardi G, Visentin R, et al. The effects of parachlorophenylalanine and naloxone on acupuncture and electroacupuncture modulation of capsaicin-induced neurogenic edema in the rat hind paw: a controlled blind study. Clin Exp Rheumatol. 1999;17:655-62. [PubMed]
- Wang DW, Zhou RB, Yao YM. Role of cholinergic anti-inflammatory pathway in regulating host response and its interventional strategy for inflammatory diseases. Chin J Traumatol 2009;12:355-64. [PubMed]
- Kavoussi B, Ross BE. The neuroimmune basis of anti-inflammatory acupuncture. Integr Cancer Ther 2007;6:251-7. [Crossref] [PubMed]
- Borovikova LV, Ivanova S, Zhang M, et al. Vagus nerve stimulation attenuates the systemic inflammatory response to endotoxin. Nature 2000;405:458-62. [Crossref] [PubMed]
- Guo ZL, Li M, Longhurst JC. Nucleus ambiguus cholinergic neurons activated by acupuncture: relation to enkephalin. Brain Res 2012;1442:25-35. [Crossref] [PubMed]
- Tsai MC, Wang YH. Effect of laser stimulation of acupoint Taichong (LR3) on blood pressure and heart rate variability. Internet Scientific Publications 2014;9:7.
- Kim DH, Ryu Y, Hahm DH, et al. Acupuncture points can be identified as cutaneous neurogenic inflammatory spots. Sci Rep 2017;7:15214. [Crossref] [PubMed]
- Kim MH, Park YC, Namgung U. Acupuncture-Stimulated Activation of Sensory Neurons. J Acupunct Meridian Stud 2012;5:148-55. [Crossref] [PubMed]
- Jindra NM, Goddard D, Imholte M, et al. Epidermal laser stimulation of action potentials in the frog sciatic nerve. J Biomed Opt 2010;15:015002. [Crossref] [PubMed]
- Wells J, Kao C, Konrad P, et al. Biophysical mechanisms responsible for pulsed low-level laser excitation of neural tissue. Proc. SPIE 6084 Optical Interactions with Tissue and Cells 2006;XVII:60840X. [Crossref]
- Miller KE, Muth ER. Efficacy of acupressure and acustimulation bands for the prevention of motion sickness. Aviat Space Environ Med 2004;75:227-34. [PubMed]
- Hentzen C, Haddad R, Sheikh Ismaël S, et al. Efficacy of posterior tibial nerve stimulation (PTNS) on overactive bladder in older adults. Eur Geriatr Med 2018;9:249-53. [Crossref] [PubMed]
- Chou DE, Shnayderman Yugrakh M, Winegarner D, et al. Acute migraine therapy with external trigeminal neurostimulation (ACME): A randomized controlled trial. Cephalalgia 2019;39:3-14. [Crossref] [PubMed]
Cite this article as: Dorsher PT, da Silva MAH. Acupuncture’s neuroanatomic and neurophysiologic basis. Longhua Chin Med 2022;5:8.